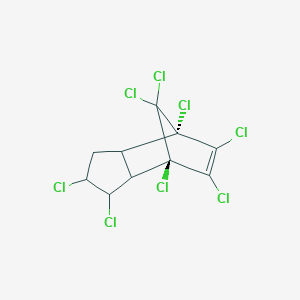
Chlordane
Overview
Description
Chlordane, also known as octachlorohexahydromethanoindene, is an organochlorine compound that was widely used as a pesticide. It is a white solid with a slightly pungent, chlorine-like odor. This compound was extensively used in agriculture and for termite treatment in buildings until it was banned in many countries due to its environmental persistence and potential health hazards .
Preparation Methods
Chlordane is synthesized by the chlorination of chlordene (hexachlorotetrahydromethanoindene), a cyclodiene compound. The industrial production of this compound involves the chlorination of chlordene in the presence of a catalyst, typically ferric chloride, at elevated temperatures. The reaction yields a mixture of cis- and trans-chlordane isomers, along with other related compounds .
Chemical Reactions Analysis
Chlordane undergoes several types of chemical reactions, including:
Oxidation: this compound can be oxidized to form various metabolites, including oxythis compound.
Reduction: Reduction of this compound can lead to the formation of less chlorinated derivatives.
Substitution: this compound can undergo nucleophilic substitution reactions, where chlorine atoms are replaced by other nucleophiles. Common reagents used in these reactions include oxidizing agents like potassium permanganate and reducing agents like lithium aluminum hydride. .
Scientific Research Applications
Chlordane has been used in various scientific research applications, including:
Chemistry: As a model compound for studying the behavior of organochlorine pesticides in the environment.
Biology: Investigating the effects of persistent organic pollutants on wildlife and ecosystems.
Medicine: Studying the toxicological effects of this compound exposure on human health.
Industry: Historically used as a pesticide for controlling termites and other pests in agricultural and urban settings
Mechanism of Action
Chlordane exerts its effects primarily by disrupting the nervous system of insects. It acts on the gamma-aminobutyric acid (GABA) receptor, inhibiting the normal function of this neurotransmitter. This leads to uncontrolled neuronal firing, resulting in paralysis and death of the insect. In mammals, this compound can cause similar neurotoxic effects, as well as liver damage and potential carcinogenicity .
Comparison with Similar Compounds
Chlordane belongs to the class of cyclodiene insecticides, which also includes compounds like heptachlor, aldrin, dieldrin, and endrin. These compounds share similar chemical structures and modes of action but differ in their environmental persistence and toxicity. This compound is unique in its high stability and long-lasting effects, making it particularly effective as a pesticide but also more hazardous in terms of environmental and health impacts .
Similar Compounds
- Heptachlor
- Aldrin
- Dieldrin
- Endrin
This compound’s persistence and bioaccumulation potential make it a significant environmental pollutant, leading to its ban in many countries .
Biological Activity
Chlordane is an organochlorine compound that was widely used as a pesticide until it was banned in many countries due to its persistence in the environment and potential health risks. This article explores the biological activity of this compound, focusing on its toxicokinetics, metabolic pathways, health effects, and epidemiological studies.
Toxicokinetics of this compound
This compound undergoes complex metabolic processes in living organisms. Its toxicokinetics involve absorption, distribution, metabolism, and excretion (ADME), which can vary significantly between species.
- Absorption : this compound can be absorbed through inhalation, dermal contact, and ingestion. Studies have shown that it is rapidly absorbed into the bloodstream after exposure .
- Distribution : Once absorbed, this compound is distributed throughout various tissues, with a notable accumulation in adipose tissue due to its lipophilic nature. The half-lives of its isomers vary; for example, cis-chlordane has a half-life of approximately 5.92 days, while nonachlor can last up to 54.1 days in biological systems .
- Metabolism : The primary metabolic pathways for this compound include:
- Excretion : this compound and its metabolites are primarily excreted through feces and urine. The rate of elimination can be influenced by factors such as age, sex, and species .
Health Effects
This compound's biological activity is associated with various adverse health effects. Below are key findings from animal studies and human epidemiological data:
Animal Studies
- Neurotoxicity : In animal models, this compound has been shown to cause neurotoxic effects. For example, prolonged exposure in rats led to increased liver cytochrome P-450 content and alterations in hematological parameters such as increased leukocyte counts .
- Carcinogenicity : Chronic exposure to this compound has been linked to the development of liver tumors in mice. Although the U.S. Department of Health and Human Services has not classified this compound as a carcinogen, several studies indicate a potential association with cancers such as non-Hodgkin's lymphoma and pancreatic cancer .
- Reproductive Effects : Studies have reported reproductive toxicity in mice exposed to this compound during gestation, including reduced size of seminiferous tubules and degeneration of spermatogenic epithelium .
Human Epidemiological Studies
Recent studies have highlighted the potential health risks associated with this compound exposure in humans:
- Diabetes Risk : A systematic review indicated that exposure to this compound compounds correlates with an increased risk of diabetes among adults. Specifically, oxythis compound and trans-nonachlor showed statistically significant associations with diabetes-related features .
- Neuropsychological Effects : Case studies involving individuals exposed to this compound through residential pest control revealed cognitive deficits and emotional distress. Symptoms included difficulties in processing speed, problem-solving abilities, and memory retention .
Data Table: Summary of Health Effects from this compound Exposure
Study Type | Organism | Exposure Duration | Key Findings |
---|---|---|---|
Animal Study | Rats | 90 days | Increased leukocyte count; hepatic effects observed |
Animal Study | Mice | Chronic | Liver tumors; reproductive toxicity noted |
Human Case Study | Humans | Subacute | Neuropsychological dysfunction post-exposure |
Epidemiological | Adults | Cross-sectional | Increased odds of diabetes with higher this compound levels |
Q & A
Basic Research Questions
Q. What are the most reliable analytical methods for quantifying chlordane in environmental and biological matrices?
- Methodological Answer : Gas chromatography with electron capture detection (GC-ECD) and gas chromatography-mass spectrometry (GC-MS) are foundational techniques for this compound quantification. For complex environmental samples, high-resolution GC combined with negative ionization mass spectrometry (HRGC-NIMS) can resolve over 120 technical this compound components, enabling precise isomer identification . In biological matrices (e.g., blood, urine), dedicated analyses should include lipid-adjusted measurements due to this compound’s lipophilicity, with oxythis compound as a primary biomarker in serum .
Q. How should researchers design experiments to investigate this compound’s toxicokinetics in mammalian models?
- Methodological Answer : Use radiolabeled trans- and cis-chlordane isomers in oral or dermal exposure studies to track absorption, distribution, and metabolism. Prioritize adipose tissue, liver, and brain sampling, as these organs accumulate this compound at higher concentrations. Repeated dosing protocols (e.g., 30-day subchronic exposure in rats) are critical to assess bioaccumulation patterns .
Q. What biomarkers are indicative of acute vs. chronic this compound exposure in humans?
- Methodological Answer : Acute exposure biomarkers include plasma this compound isomers (trans-chlordane, cis-chlordane) and heptachlor epoxide, measurable via GC-MS. For chronic exposure, monitor oxythis compound in serum and adipose tissue, which persists due to slow metabolic degradation. Hematological analyses (e.g., lymphocyte counts) may supplement biomarker data but require correlation with exposure histories .
Advanced Research Questions
Q. How can researchers resolve discrepancies in this compound component analysis caused by environmental degradation or matrix interference?
- Methodological Answer : Apply HRGC with dual-column retention indices (e.g., DB-5 and DB-1701 stationary phases) to distinguish degradation products like chlorobornanes. Use electron capture negative ion mass spectrometry (ECNIMS) to identify structural features of non-target components. For quantitation, report the sum of all identifiable isomers as "this compound (n.o.s.)" under CAS 57-74-9 to standardize data across studies .
Q. What methodologies are recommended for assessing long-term environmental trends of this compound in Arctic ecosystems?
- Methodological Answer : Analyze temporal trends using enantiomer fractions (EFs) of trans-chlordane and cis-chlordane in air samples. Non-racemic EFs (e.g., EF > 0.5) indicate contributions from weathered soil residues rather than fresh emissions. Combine isomer-specific data (e.g., trans-nonachlor ratios) with passive air sampling to model long-range transport mechanisms .
Q. How can microarray data improve the interpretation of this compound’s nongenotoxic hepatocarcinogenicity?
- Methodological Answer : Use toxicogenomic approaches to identify gene expression signatures in mouse liver tumors. Collaborate with bioinformatics experts to analyze pathways linked to oxidative stress (e.g., CYP450 upregulation) and cell proliferation. Validate findings with immunohistochemistry for proteins like Ki-67 to confirm proliferative indices .
Q. What strategies address data gaps in this compound’s interactions with other organochlorines during risk assessment?
- Methodological Answer : Conduct mixture toxicity studies using factorial experimental designs to evaluate synergistic effects (e.g., this compound + DDT). Prioritize in vitro assays (e.g., human hepatocyte cultures) to assess cytochrome P450 inhibition. Integrate physiologically based pharmacokinetic (PBPK) modeling to extrapolate cross-species interactions .
Q. Methodological Challenges and Contradictions
Q. How should conflicting reports on this compound’s carcinogenic potential be reconciled?
- Methodological Answer : Discrepancies arise from variations in exposure matrices (e.g., technical this compound vs. purified isomers) and model organisms. Address this by standardizing test materials using ATSDR’s peer-reviewed inclusion criteria (Table B-1) and prioritizing studies with dose-response data. Cross-validate epidemiological findings (e.g., non-Hodgkin’s lymphoma links) with mechanistic in vitro mutagenicity assays .
Q. What frameworks are recommended for prioritizing this compound research under CERCLA Section 104(i)(5)?
- Methodological Answer : Follow ATSDR’s two-step literature screening process: (1) Title/abstract filtering using terms like “biomarkers,” “toxicokinetics,” and “susceptible populations”; (2) Full-text review by multidisciplinary panels to eliminate bias. Prioritize studies on endocrine disruption and transplacental transfer, which are underrepresented in current databases .
Q. Data Interpretation Guidelines
Q. How should researchers interpret non-racemic enantiomer fractions in this compound environmental samples?
- Methodological Answer : Non-racemic EFs (e.g., EF = 0.66 for trans-chlordane) indicate enantioselective microbial degradation in soils. Compare EFs across matrices (air, water, sediment) to differentiate recent vs. historic emissions. Use chiral columns (e.g., β-cyclodextrin) for enantiomer separation and confirm findings with soil emission flux studies .
Q. What statistical approaches are optimal for analyzing this compound’s heterogeneous occurrence in drinking water systems?
- Methodological Answer : Apply spatial interpolation models (e.g., kriging) to datasets like the EPA’s SYR 4 ICR (Exhibit B-14). Use Monte Carlo simulations to estimate exposure thresholds below practical quantitation limits (PQLs). Report geometric means and 95th percentiles to account for log-normal distribution in contamination levels .
Properties
CAS No. |
57-74-9 |
---|---|
Molecular Formula |
C10H6Cl8 |
Molecular Weight |
409.8 g/mol |
IUPAC Name |
(1R,7S)-1,3,4,7,8,9,10,10-octachlorotricyclo[5.2.1.02,6]dec-8-ene |
InChI |
InChI=1S/C10H6Cl8/c11-3-1-2-4(5(3)12)9(16)7(14)6(13)8(2,15)10(9,17)18/h2-5H,1H2/t2?,3?,4?,5?,8-,9+/m0/s1 |
InChI Key |
BIWJNBZANLAXMG-YQELWRJZSA-N |
impurities |
Several new components of technical chlordane were discovered using electron capture, negative ionization gas chromatographic mass spectrometry. These compounds have 10-12 chlorines and are produced by the condensation of three cyclopentadiene molecules. The most abundant compound has a molecular weight of 606 and has the elemental composition C15H6Cl12. This compound and a series of related compounds were also identified as contaminants in human adipose tissue samples. These compounds are approximately 0.01-0.03% of the technical chlordane mixture, and they have average concentrations in human adipose tissue of 0.4-0.7 ng/g of fat. They are more highly retained in human adipose tissue than chlordane-like compounds containing eight or fewer chlorine atoms. |
SMILES |
C1C2C(C(C1Cl)Cl)C3(C(=C(C2(C3(Cl)Cl)Cl)Cl)Cl)Cl |
Isomeric SMILES |
C1C2C(C(C1Cl)Cl)[C@]3(C(=C([C@@]2(C3(Cl)Cl)Cl)Cl)Cl)Cl |
Canonical SMILES |
C1C2C(C(C1Cl)Cl)C3(C(=C(C2(C3(Cl)Cl)Cl)Cl)Cl)Cl |
boiling_point |
175 °C @ 1 mm Hg at 0.27kPa: 175 °C decomposes Decomposes |
Color/Form |
Viscous, amber-colored liquid Colorless, viscous liquid White crystals |
density |
1.59-1.63 @ 25 °C Relative density (water = 1): 1.59 - 1.63 1.56 (77 °F): 1.6 |
flash_point |
Solution: 225 °F (open cup), 132 °F (closed cup) |
melting_point |
Melting point: 106-107 °C cis-isomer; 104-105 °C trans-isomer 217-228 °F |
Key on ui other cas no. |
57-74-9 |
physical_description |
Amber-colored, viscous liquid with a pungent, chlorine-like odor. [insecticide]; [NIOSH] Solid; [Cerilliant MSDS] White odorless crystals; [Sigma-Aldrich MSDS] TECHNICAL-GRADE PRODUCT: LIGHT YELLOW-TO-AMBER VISCOUS LIQUID. Amber-colored, viscous liquid with a pungent, chlorine-like odor. Amber-colored, viscous liquid with a pungent, chlorine-like odor. [insecticide] |
Pictograms |
Irritant; Health Hazard; Environmental Hazard |
shelf_life |
Dehydrohalogenates in presence of alkali |
solubility |
Miscible with aliphatic and aromatic hydrocarbon solvents, including deodorized kerosene In water, 0.056 mg/L @ 25 °C Solubility in water: none 0.0001% |
Synonyms |
Octachloro-hexahydro-methano-1H-indene |
vapor_density |
14: (air= 1 at boiling point of chlordane) 14 |
vapor_pressure |
0.00001 [mmHg] 0.000036 [mmHg] 0.0000503 [mmHg] 9.75X10-6 mm Hg @ 25 °C Vapor pressure, Pa at 25 °C: 0.0013 0.00001 mmHg |
Origin of Product |
United States |
Retrosynthesis Analysis
AI-Powered Synthesis Planning: Our tool employs the Template_relevance Pistachio, Template_relevance Bkms_metabolic, Template_relevance Pistachio_ringbreaker, Template_relevance Reaxys, Template_relevance Reaxys_biocatalysis model, leveraging a vast database of chemical reactions to predict feasible synthetic routes.
One-Step Synthesis Focus: Specifically designed for one-step synthesis, it provides concise and direct routes for your target compounds, streamlining the synthesis process.
Accurate Predictions: Utilizing the extensive PISTACHIO, BKMS_METABOLIC, PISTACHIO_RINGBREAKER, REAXYS, REAXYS_BIOCATALYSIS database, our tool offers high-accuracy predictions, reflecting the latest in chemical research and data.
Strategy Settings
Precursor scoring | Relevance Heuristic |
---|---|
Min. plausibility | 0.01 |
Model | Template_relevance |
Template Set | Pistachio/Bkms_metabolic/Pistachio_ringbreaker/Reaxys/Reaxys_biocatalysis |
Top-N result to add to graph | 6 |
Feasible Synthetic Routes
Disclaimer and Information on In-Vitro Research Products
Please be aware that all articles and product information presented on BenchChem are intended solely for informational purposes. The products available for purchase on BenchChem are specifically designed for in-vitro studies, which are conducted outside of living organisms. In-vitro studies, derived from the Latin term "in glass," involve experiments performed in controlled laboratory settings using cells or tissues. It is important to note that these products are not categorized as medicines or drugs, and they have not received approval from the FDA for the prevention, treatment, or cure of any medical condition, ailment, or disease. We must emphasize that any form of bodily introduction of these products into humans or animals is strictly prohibited by law. It is essential to adhere to these guidelines to ensure compliance with legal and ethical standards in research and experimentation.