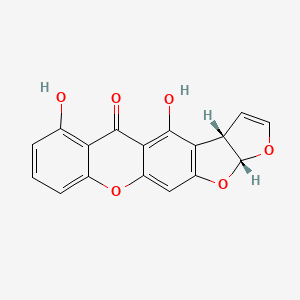
Sterigmatin
Overview
Description
Sterigmatin is a naturally occurring compound that belongs to the class of xanthones. It is a metabolite produced by the mold species Aspergillus versicolor . The compound has a complex structure, characterized by a combination of a xanthone nucleus and a bisdihydrofuran ring . This compound is known for its biological activities and has been the subject of various scientific studies.
Mechanism of Action
Target of Action
Sterigmatin is a metabolite of Aspergillus versicolor It’s known that this compound interacts with the atp synthesis system in mitochondria .
Mode of Action
this compound interacts with its targets, particularly the ATP synthesis system in mitochondria . The interaction of this compound with its targets leads to changes in the oxidative phosphorylation in mitochondria, causing marked decreases in RC index and P/O ratio .
Biochemical Pathways
this compound affects the oxidative phosphorylation pathway in mitochondria . This interaction leads to a decrease in the RC index and P/O ratio, indicating a disruption in the normal functioning of the mitochondria .
Result of Action
The interaction of this compound with the ATP synthesis system in mitochondria leads to a disruption in the normal functioning of the mitochondria . This disruption is characterized by a decrease in the RC index and P/O ratio, which are indicators of mitochondrial function .
Biochemical Analysis
Biochemical Properties
Sterigmatin plays a significant role in biochemical reactions, particularly in the oxidative phosphorylation process within mitochondria. It has been found to uncouple oxidative phosphorylation, leading to a decrease in the respiratory control index and the P/O ratio . This compound interacts with various enzymes and proteins involved in mitochondrial function, including those in the ATP synthesis system. The compound’s linear structure, combining a dihydrobisfuran ring and a xanthone nucleus, contributes to its higher toxicity compared to its angular counterpart, demethylsterigmatocystin .
Cellular Effects
This compound exerts toxic effects on various cell types, particularly hepatocytes. It has been shown to impair mitochondrial functions, leading to decreased ATP production and increased cytotoxicity . The compound’s impact on cell signaling pathways, gene expression, and cellular metabolism is significant, as it disrupts normal cellular processes and induces oxidative stress. This compound’s cytotoxicity is more pronounced in hepatocytes compared to demethylsterigmatocystin, highlighting its potent cellular effects .
Molecular Mechanism
At the molecular level, this compound exerts its effects by binding to mitochondrial enzymes and proteins, disrupting the normal function of the oxidative phosphorylation pathway . This binding leads to the uncoupling of oxidative phosphorylation, resulting in decreased ATP production and increased production of reactive oxygen species. This compound’s linear structure allows for more effective binding interactions compared to the angular structure of demethylsterigmatocystin . Additionally, this compound has been shown to induce changes in gene expression related to oxidative stress and mitochondrial dysfunction .
Temporal Effects in Laboratory Settings
In laboratory settings, the effects of this compound have been observed to change over time. The compound’s stability and degradation are critical factors influencing its long-term effects on cellular function. Studies have shown that this compound remains stable under certain conditions, but its degradation products can also exert toxic effects . Long-term exposure to this compound in in vitro and in vivo studies has demonstrated persistent mitochondrial dysfunction and cytotoxicity, indicating the compound’s lasting impact on cellular health .
Dosage Effects in Animal Models
The effects of this compound vary with different dosages in animal models. At lower doses, this compound induces mild mitochondrial dysfunction and oxidative stress, while higher doses result in severe cytotoxicity and hepatotoxicity . Threshold effects have been observed, where a certain dosage level leads to a significant increase in toxic effects. High doses of this compound can cause adverse effects, including liver damage and increased mortality in animal models .
Metabolic Pathways
This compound is involved in several metabolic pathways, particularly those related to mitochondrial function. It interacts with enzymes and cofactors involved in oxidative phosphorylation, leading to disruptions in metabolic flux and alterations in metabolite levels . The compound’s impact on mitochondrial metabolism is significant, as it impairs ATP production and increases the production of reactive oxygen species, contributing to its overall toxicity .
Transport and Distribution
Within cells and tissues, this compound is transported and distributed through interactions with specific transporters and binding proteins . These interactions influence the compound’s localization and accumulation in different cellular compartments. This compound’s distribution within cells is critical for its toxic effects, as it primarily targets mitochondria and disrupts their function .
Subcellular Localization
This compound’s subcellular localization is primarily within mitochondria, where it exerts its toxic effects . The compound’s targeting signals and post-translational modifications direct it to specific compartments within the mitochondria, allowing it to interact with key enzymes and proteins involved in oxidative phosphorylation. This localization is essential for this compound’s ability to disrupt mitochondrial function and induce cytotoxicity .
Preparation Methods
Synthetic Routes and Reaction Conditions: Sterigmatin can be synthesized through a series of chemical reactions starting from simpler organic compoundsThe reaction conditions often require the use of specific catalysts and solvents to facilitate the formation of the desired product .
Industrial Production Methods: Industrial production of this compound involves the cultivation of Aspergillus versicolor under controlled conditions. The mold is grown in a nutrient-rich medium, and the metabolites are extracted and purified using various chromatographic techniques . The process requires careful monitoring to ensure the optimal production of this compound.
Chemical Reactions Analysis
Types of Reactions: Sterigmatin undergoes several types of chemical reactions, including oxidation, reduction, and substitution reactions. These reactions are essential for modifying the compound’s structure and enhancing its biological activity .
Common Reagents and Conditions:
Oxidation: Common oxidizing agents such as potassium permanganate or hydrogen peroxide are used to introduce oxygen-containing functional groups into the this compound molecule.
Reduction: Reducing agents like sodium borohydride or lithium aluminum hydride are employed to reduce specific functional groups within the compound.
Substitution: Various nucleophiles can be used to substitute specific atoms or groups within the this compound structure.
Major Products: The major products formed from these reactions depend on the specific reagents and conditions used. For example, oxidation reactions may yield hydroxylated derivatives, while reduction reactions can produce deoxygenated forms of this compound .
Scientific Research Applications
Sterigmatin has a wide range of scientific research applications:
Chemistry: It is used as a model compound for studying the reactivity of xanthones and bisdihydrofuran rings.
Biology: this compound is studied for its effects on cellular processes and its potential as a bioactive compound.
Medicine: Research has explored its potential as an anticancer agent due to its ability to interfere with cellular metabolism.
Comparison with Similar Compounds
Sterigmatin is unique due to its linear combination of a xanthone nucleus and a bisdihydrofuran ring. Similar compounds include:
Sterigmatocystin: A related mycotoxin with a similar structure but different biological activity.
Austocystins: Metabolites of Aspergillus ustus with a linear fusion between the xanthone and bisdihydrofuran moieties.
Aflatoxin B1: Another mycotoxin with a similar biosynthetic pathway but distinct toxicological properties.
This compound’s uniqueness lies in its specific structural features and its potent biological activities, making it a valuable compound for scientific research.
Properties
IUPAC Name |
2,18-dihydroxy-7,9,13-trioxapentacyclo[10.8.0.03,10.04,8.014,19]icosa-1,3(10),5,11,14,16,18-heptaen-20-one | |
---|---|---|
Details | Computed by Lexichem TK 2.7.0 (PubChem release 2021.10.14) | |
Source | PubChem | |
URL | https://pubchem.ncbi.nlm.nih.gov | |
Description | Data deposited in or computed by PubChem | |
InChI |
InChI=1S/C17H10O6/c18-8-2-1-3-9-13(8)16(20)14-11(22-9)6-10-12(15(14)19)7-4-5-21-17(7)23-10/h1-7,17-19H | |
Details | Computed by InChI 1.0.6 (PubChem release 2021.10.14) | |
Source | PubChem | |
URL | https://pubchem.ncbi.nlm.nih.gov | |
Description | Data deposited in or computed by PubChem | |
InChI Key |
CWYJYLXZMAUSNI-UHFFFAOYSA-N | |
Details | Computed by InChI 1.0.6 (PubChem release 2021.10.14) | |
Source | PubChem | |
URL | https://pubchem.ncbi.nlm.nih.gov | |
Description | Data deposited in or computed by PubChem | |
Canonical SMILES |
C1=CC(=C2C(=C1)OC3=CC4=C(C5C=COC5O4)C(=C3C2=O)O)O | |
Details | Computed by OEChem 2.3.0 (PubChem release 2021.10.14) | |
Source | PubChem | |
URL | https://pubchem.ncbi.nlm.nih.gov | |
Description | Data deposited in or computed by PubChem | |
Molecular Formula |
C17H10O6 | |
Details | Computed by PubChem 2.2 (PubChem release 2021.10.14) | |
Source | PubChem | |
URL | https://pubchem.ncbi.nlm.nih.gov | |
Description | Data deposited in or computed by PubChem | |
DSSTOX Substance ID |
DTXSID10970588 | |
Record name | 4,6-Dihydroxy-3a,12a-dihydro-5H-furo[3',2':4,5]furo[3,2-b]xanthen-5-one | |
Source | EPA DSSTox | |
URL | https://comptox.epa.gov/dashboard/DTXSID10970588 | |
Description | DSSTox provides a high quality public chemistry resource for supporting improved predictive toxicology. | |
Molecular Weight |
310.26 g/mol | |
Details | Computed by PubChem 2.2 (PubChem release 2021.10.14) | |
Source | PubChem | |
URL | https://pubchem.ncbi.nlm.nih.gov | |
Description | Data deposited in or computed by PubChem | |
CAS No. |
55256-49-0 | |
Record name | 4,6-Dihydroxy-3a,12a-dihydro-5H-furo[3',2':4,5]furo[3,2-b]xanthen-5-one | |
Source | EPA DSSTox | |
URL | https://comptox.epa.gov/dashboard/DTXSID10970588 | |
Description | DSSTox provides a high quality public chemistry resource for supporting improved predictive toxicology. | |
Retrosynthesis Analysis
AI-Powered Synthesis Planning: Our tool employs the Template_relevance Pistachio, Template_relevance Bkms_metabolic, Template_relevance Pistachio_ringbreaker, Template_relevance Reaxys, Template_relevance Reaxys_biocatalysis model, leveraging a vast database of chemical reactions to predict feasible synthetic routes.
One-Step Synthesis Focus: Specifically designed for one-step synthesis, it provides concise and direct routes for your target compounds, streamlining the synthesis process.
Accurate Predictions: Utilizing the extensive PISTACHIO, BKMS_METABOLIC, PISTACHIO_RINGBREAKER, REAXYS, REAXYS_BIOCATALYSIS database, our tool offers high-accuracy predictions, reflecting the latest in chemical research and data.
Strategy Settings
Precursor scoring | Relevance Heuristic |
---|---|
Min. plausibility | 0.01 |
Model | Template_relevance |
Template Set | Pistachio/Bkms_metabolic/Pistachio_ringbreaker/Reaxys/Reaxys_biocatalysis |
Top-N result to add to graph | 6 |
Feasible Synthetic Routes
Q1: What is the mechanism by which Sterigmatin exerts its toxic effects on cells?
A1: this compound exhibits its toxicity primarily by disrupting mitochondrial function. It acts as an uncoupler of oxidative phosphorylation in rat liver mitochondria, leading to a decrease in the respiratory control index (RC index) and the P/O ratio []. This uncoupling effect essentially means that this compound disrupts the mitochondria's ability to efficiently produce ATP, the cell's primary energy currency, ultimately compromising cellular function.
Q2: How does the structure of this compound compare to its related compound, Demethylsterigmatocystin, and how do these structural differences affect their biological activity?
A2: this compound and Demethylsterigmatocystin are structurally similar, both belonging to the sterigmatocystin family of mycotoxins. The key difference lies in the fusion of the xanthone and bisdihydrofuran moieties. In this compound, these moieties are fused linearly, while in Demethylsterigmatocystin, the fusion is angular []. This structural variation significantly impacts their uncoupling activity on mitochondria, with the linearly structured this compound exhibiting higher toxicity compared to the angular Demethylsterigmatocystin [].
Q3: What is the role of this compound in the biosynthesis of Aflatoxins?
A3: this compound plays a crucial role as a precursor in the intricate biosynthetic pathway of aflatoxins, specifically aflatoxin B1 (AFB1) and aflatoxin G1 (AFG1) []. It is derived from Versicolorin A, a common precursor to several mycotoxins including sterigmatocystin and aflatoxin B1 []. Research suggests that the absolute configuration of the bisdihydrofuran moiety in this compound is identical to that in sterigmatocystin and aflatoxin B1, highlighting the shared biosynthetic lineage [].
Q4: Are there any known enzymatic transformations involving this compound within the aflatoxin biosynthetic pathway?
A4: Yes, studies using Aspergillus parasiticus NIAH-26, a mutant strain incapable of producing aflatoxins or their precursors, revealed that feeding it with Demethylsterigmatocystin resulted in the production of aflatoxins B1 and G1 []. This suggests the involvement of specific O-methyltransferases (enzymes that catalyze the transfer of a methyl group) in converting Demethylsterigmatocystin to Sterigmatocystin and subsequently to O-methylsterigmatocystin, a direct precursor to aflatoxins []. Interestingly, these O-methyltransferases exhibit substrate specificity and are differentially affected by inhibitors, indicating a complex regulatory mechanism within the pathway [].
Q5: What are the implications of this compound and related compounds for food safety?
A5: this compound, like other members of the sterigmatocystin family, is a secondary metabolite produced by certain Aspergillus species commonly found in grains and stored foods []. Its presence raises significant food safety concerns due to its potential toxicity. Analytical techniques like high-speed liquid chromatography have been developed to detect and quantify this compound and related metabolites in food products [], enabling better monitoring and mitigation strategies to ensure consumer safety.
Q6: What spectroscopic data can be used to characterize this compound?
A6: this compound can be characterized using various spectroscopic techniques. It has a molecular formula of C17H10O6 and a molecular weight of 310 g/mol []. Ultraviolet-visible (UV) spectroscopy reveals a characteristic absorption maximum at 243 nm []. Further structural insights can be obtained from infrared (IR) spectroscopy and nuclear magnetic resonance (NMR) spectroscopy, providing information about functional groups and the arrangement of atoms within the molecule. Additionally, mass spectrometry (MS) can be employed to determine the molecular weight and fragmentation pattern of this compound, aiding in its identification and characterization.
Q7: What is known about the crystal structure of this compound?
A7: The crystal structure of this compound has been elucidated using single-crystal X-ray analysis []. It crystallizes in the triclinic space group P1, with two crystallographically independent molecules in the unit cell []. The structure reveals two intramolecular hydrogen bonds within each molecule, contributing to its overall stability and conformation []. This detailed structural information provides valuable insights into the molecular interactions and properties of this compound.
Disclaimer and Information on In-Vitro Research Products
Please be aware that all articles and product information presented on BenchChem are intended solely for informational purposes. The products available for purchase on BenchChem are specifically designed for in-vitro studies, which are conducted outside of living organisms. In-vitro studies, derived from the Latin term "in glass," involve experiments performed in controlled laboratory settings using cells or tissues. It is important to note that these products are not categorized as medicines or drugs, and they have not received approval from the FDA for the prevention, treatment, or cure of any medical condition, ailment, or disease. We must emphasize that any form of bodily introduction of these products into humans or animals is strictly prohibited by law. It is essential to adhere to these guidelines to ensure compliance with legal and ethical standards in research and experimentation.