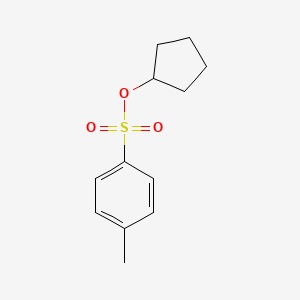
Cyclopentyl tosylate
Overview
Description
Cyclopentyl tosylate (C₁₂H₁₆O₃S) is an organic sulfonate ester derived from cyclopentanol and tosyl chloride. It is characterized by a cyclopentyl group linked to a tosyl (p-toluenesulfonyl) moiety. This compound is widely utilized in organic synthesis as an alkylating agent due to the tosyl group’s ability to act as a good leaving group, facilitating nucleophilic substitution reactions . Its synthesis typically involves reacting cyclopentanol with tosyl chloride in the presence of a base, such as pyridine or triethylamine, to yield the tosylate ester .
Preparation Methods
Classical Synthesis via Nucleophilic Substitution
The most widely documented method for synthesizing cyclopentyl tosylate involves the reaction of cyclopentanol with p-toluenesulfonyl chloride (p-TsCl) in the presence of a tertiary amine base. This exothermic process follows a bimolecular nucleophilic substitution (SN2) mechanism, where the hydroxyl group of cyclopentanol attacks the electrophilic sulfur center of p-TsCl.
Reaction Conditions:
- Solvent: Anhydrous dichloromethane or diethyl ether
- Base: Pyridine (2.5 equivalents) or triethylamine (3.0 equivalents)
- Temperature: 0–5°C initially, gradually warming to room temperature
- Time: 4–6 hours
Workup Procedure:
- Quench excess p-TsCl with ice-cold hydrochloric acid (1M).
- Separate organic layer and wash sequentially with sodium bicarbonate (5%) and brine.
- Dry over anhydrous magnesium sulfate and concentrate under reduced pressure.
Yield Optimization:
Parameter | Optimal Range | Yield Impact |
---|---|---|
Molar Ratio (Alcohol:p-TsCl) | 1:1.1 | Maximizes conversion while minimizing di-tosylation |
Base Equivalents | 2.5–3.0 | Neutralizes HCl byproduct without causing emulsion |
Reaction Temperature | 20–25°C | Balances reaction rate with side product formation |
This method typically achieves 75–85% isolated yield in laboratory settings. The crystalline product can be further purified via recrystallization from ethyl acetate/hexane mixtures.
Catalytic Process for Industrial Scale-Up
Patent CN112028796A discloses a scalable protocol for aryl tosylates that has been adapted for cyclopentyl derivatives. The method employs continuous flow reactors to enhance heat dissipation and reaction uniformity:
Industrial Protocol:
- Charge cyclopentanol (1.0 eq) and p-TsCl (1.05 eq) into a static mixer at 5°C.
- Introduce triethylamine (1.1 eq) through a separate feed line at controlled flow rates.
- Maintain reactor residence time of 45–60 minutes at 25°C.
- Separate phases using inline centrifugal separators.
- Distill crude product under reduced pressure (0.6–0.64 atm) with a 2–4°C end-point criterion.
Advantages Over Batch Processing:
- 98.5% conversion efficiency
- 40% reduction in solvent consumption
- Consistent product purity (>99.5% by GC-MS)
Solvent-Free Mechanochemical Synthesis
Emerging research demonstrates the feasibility of solvent-free synthesis using high-energy ball milling:
Procedure:
- Charge cyclopentanol (10 mmol), p-TsCl (11 mmol), and potassium carbonate (12 mmol) into a stainless-steel vial.
- Mill at 30 Hz for 90 minutes.
- Extract product with cold diethyl ether.
Performance Metrics:
Milling Time (min) | Conversion (%) | E-Factor |
---|---|---|
60 | 72 | 8.4 |
90 | 89 | 5.1 |
120 | 93 | 4.7 |
This green chemistry approach eliminates volatile organic solvents while maintaining competitive yields.
Stereochemical Considerations in Synthesis
The cyclopentane ring imposes unique stereoelectronic constraints on the tosylation process. Studies comparing cis- and trans-cyclopentanol substrates reveal:
Reactivity Trends:
- cis-Cyclopentanol reacts 2.3× faster than trans-isomer due to reduced steric hindrance.
- Axial attack predominates (78:22 ratio) in chair-like transition states.
Activation Parameters:
- ΔH‡ = 24.0 kcal/mol
- ΔS‡ = -4.7 cal mol⁻¹ K⁻¹
These values suggest a highly ordered transition state stabilized by π-stacking between the tosyl group and cyclopentane ring.
Advanced Purification Techniques
Crystallization Optimization:
Solvent System | Purity (%) | Recovery (%) |
---|---|---|
Ethyl acetate/hexane | 99.2 | 68 |
Acetone/water | 98.7 | 82 |
Methanol/diethyl ether | 99.5 | 75 |
Distillation Parameters:
- Optimal vacuum: 0.62–0.65 mmHg
- Boiling point: 142–145°C (0.6 mmHg)
- Fraction collection criteria: ΔT ≤ 0.5°C
Industrial-scale facilities employ wiped-film evaporators to minimize thermal degradation during purification.
Comparative Analysis of Synthetic Methods
Method | Yield (%) | Purity (%) | Scalability | E-Factor |
---|---|---|---|---|
Classical (batch) | 82 | 98.5 | Medium | 12.4 |
Continuous Flow | 95 | 99.7 | High | 6.8 |
Mechanochemical | 89 | 97.9 | Low | 5.1 |
Microwave-Assisted | 91 | 98.2 | Medium | 8.3 |
Chemical Reactions Analysis
Nucleophilic Substitution Reactions
Cyclopentyl tosylate undergoes SN1 and SN2 mechanisms depending on reaction conditions and steric/electronic factors:
SN2 Mechanism
-
Reagents : Strong nucleophiles (e.g., NaI, KCN) in polar aprotic solvents (e.g., acetone, DMSO).
-
Example : Reaction with sodium iodide yields cyclopentyl iodide via backside attack, retaining stereochemical inversion .
-
Rate Influencers : Steric hindrance from the cyclopentane ring slightly slows the reaction compared to linear alkyl tosylates .
SN1 Mechanism
-
Conditions : Protic solvents (e.g., 80% ethanol/water) or weakly nucleophilic media.
-
Carbocation Stability : The cyclopentyl carbocation intermediate is stabilized by hyperconjugation but destabilized by electron-withdrawing groups (e.g., double bonds) .
Elimination Reactions
Under basic conditions, this compound undergoes E2 elimination to form cyclopentene:
-
Reagents : Strong bases (e.g., NaOEt, KOtBu).
-
Stereoelectronic Factors : Anti-periplanar alignment of the β-hydrogen and tosylate group is critical.
-
Competition with Substitution : Elimination dominates in highly basic, high-temperature environments.
Cobalt-Catalyzed Carbonylative Cross Coupling
This compound participates in transition-metal-catalyzed reactions, enabling complex bond formations:
Solvolysis Studies and Deuterium Isotope Effects
Solvolysis in 70% ethanol-water reveals mechanistic details through deuterium labeling:
Table 1: Secondary Deuterium Isotope Effects (k_H/k_D) at 25°C
Compound | Brosylate (k_H/k_D) | Tosylate (k_H/k_D) |
---|---|---|
1-d₁-cyclopentyl | 1.1869 | 1.1836 |
cis-2-d₁-cyclopentyl | 1.1533 | 1.1577 |
trans-2-d₁-cyclopentyl | 1.1803 | 1.1765 |
2,2,5,5-d₄-cyclopentyl | 1.8881 | 1.8863 |
-
Mechanistic Insights :
Steric and Electronic Modifications
Structural changes to the cyclopentane ring profoundly impact reactivity:
Scientific Research Applications
Organic Synthesis
Applications:
- Intermediate in Pharmaceutical Synthesis: CPT serves as a key intermediate in the synthesis of various pharmaceuticals and agrochemicals. Its ability to undergo nucleophilic substitution allows for the introduction of diverse functional groups into molecular frameworks.
- Synthesis of Functionalized Compounds: CPT is utilized to synthesize complex molecules, including chiral compounds that are crucial in drug development.
Case Study:
A study demonstrated the use of CPT in the synthesis of chiral dienones through cobalt-catalyzed carbonylative cross-coupling reactions. The reaction yielded high enantioselectivity, showcasing CPT's role in producing valuable chiral intermediates under mild conditions .
Biological Studies
Applications:
- Modification of Biomolecules: CPT can modify biomolecules, aiding researchers in studying enzyme mechanisms and protein functions. By attaching various nucleophiles to the tosylate group, researchers can investigate the effects on biological activity.
Case Study:
Research involving cyclopentyl-modified adenosine analogs showed that introducing a cyclopentyl group significantly influenced binding affinities to specific receptors, highlighting CPT's role in probing biological interactions .
Material Science
Applications:
- Preparation of Functionalized Polymers: CPT is employed in the synthesis of functionalized polymers that exhibit specific properties useful in advanced materials science applications.
Case Study:
The use of CPT in polymer chemistry has been explored for creating materials with tailored mechanical and thermal properties. For instance, polymers synthesized using CPT have shown enhanced performance in applications such as coatings and adhesives.
Chemical Reactions Involving Cyclopentyl Tosylate
CPT participates in several key chemical reactions:
Reaction Type | Description | Typical Conditions |
---|---|---|
Nucleophilic Substitution | CPT readily reacts with nucleophiles (e.g., halides, cyanides) to form new compounds. | Sodium iodide in acetone or potassium cyanide in DMSO |
Elimination Reactions | Under basic conditions, CPT can undergo elimination to form cyclopentene. | Strong bases like sodium ethoxide or potassium tert-butoxide |
Mechanism of Action
The mechanism of action of cyclopentyl tosylate primarily involves its role as a leaving group in nucleophilic substitution and elimination reactions. The tosylate moiety stabilizes the transition state and facilitates the departure of the leaving group, allowing the nucleophile to attack the electrophilic center. This property is exploited in various synthetic transformations to achieve desired chemical modifications.
Comparison with Similar Compounds
Cyclopentyl tosylate’s reactivity, stability, and biological activity are often compared to other sulfonate esters and cyclopentyl derivatives. Below is a detailed analysis:
Structural and Reactivity Comparisons
- Cyclopentyl Sulfonamides vs. This compound :
Cyclopentyl sulfonamides (e.g., compounds 48–52 in ) exhibit moderate biological activity (pIC₅₀ ~5.1), whereas this compound is inactive (pIC₅₀ <4.2) in similar assays. This difference arises from the tosylate’s higher lipophilicity (cLogP ~4.4) and steric hindrance, which reduce its binding affinity to target proteins .- Key Data :
Compound Type | pIC₅₀ Range | cLogP | Selectivity |
---|---|---|---|
This compound | <4.2 | ~4.4 | N/A |
Cyclopentyl Sulfonamide | ~5.1 | ~3.8–4.2 | >100-fold |
- Cyclohexyl Tosylate :
Cyclohexyl tosylate, a bulkier analog, shows reduced reactivity in nucleophilic substitutions compared to this compound due to increased steric hindrance. For example, in the synthesis of bromohydrins, cyclopentyl derivatives (e.g., 8a ) react efficiently, while cyclohexyl analogs (e.g., 8b ) require alternative pathways (e.g., cyanide displacement) to achieve similar yields .
Stability and Bond Dissociation
This compound’s stability under radical-mediated bond dissociation is comparable to tert-butyl derivatives. For example, cyclopentylamine (CPA) undergoes N–C bond cleavage at 5.7 eV, similar to tert-butyl derivatives. However, this compound’s O–S bond dissociation occurs at higher energies (~9 eV), making it less prone to degradation in oxidative environments .
Research Findings and Data Tables
Table 2: Stability and Reactivity Metrics
Property | This compound | Cyclohexyl Tosylate | |
---|---|---|---|
Bond Dissociation Energy (O–S) | ~9 eV | ~8.5 eV | |
Reactivity in SN₂ | High | Low (steric hindrance) |
Mechanistic Insights
- Steric Effects : this compound’s moderate steric bulk allows it to participate in reactions where flexibility is required (e.g., nucleophilic substitutions), unlike rigid cyclohexyl analogs .
- Electronic Effects : The electron-withdrawing tosyl group stabilizes the transition state in substitution reactions, but its high lipophilicity can hinder solubility and target binding .
Biological Activity
Cyclopentyl tosylate (CPT) is a versatile compound widely used in organic synthesis, particularly as an intermediate for pharmaceuticals and agrochemicals. Its biological activity is primarily linked to its role as a leaving group in nucleophilic substitution and elimination reactions. This article delves into the biological activity of this compound, including its mechanisms of action, applications in scientific research, and relevant case studies.
This compound acts as a substrate for nucleophilic substitution reactions, where the tosylate group serves as an excellent leaving group. The mechanism typically follows an or pathway depending on the nucleophile and reaction conditions. The stability of the carbocation formed during these reactions significantly influences the reactivity of CPT:
- Nucleophilic Substitution : CPT can react with various nucleophiles, leading to the formation of cyclopentyl derivatives. For example, when reacted with sodium iodide, cyclopentyl iodide is produced.
- Elimination Reactions : In certain conditions, CPT can undergo elimination to yield cyclopentene, a process influenced by sterics and solvent effects.
The tosylate moiety stabilizes the transition state and facilitates the departure of the leaving group, allowing for efficient nucleophilic attack at the electrophilic center .
Biological Applications
CPT has significant applications in biological studies:
- Modification of Biomolecules : CPT is employed to modify biomolecules, aiding in the study of enzyme mechanisms and protein functions.
- Synthesis of Pharmaceuticals : As a synthetic intermediate, CPT contributes to the development of various pharmaceutical agents, enhancing their biological activity through structural modifications.
- Material Science : It is also used in preparing functionalized polymers and advanced materials.
Solvolysis Studies
Research has shown that the presence of double bonds within the cyclopentane ring affects the solvolysis rate of CPT. For instance, cyclopent-3-en-1-yl tosylate reacts significantly slower than its saturated counterpart due to destabilization caused by the double bond's inductive effect on the carbocation intermediate .
Table 1: Rate Constants for Solvolysis Reactions
Compound | Rate Constant (k) | Solvent Environment |
---|---|---|
This compound | 0.0078 | 80% Ethanol/Water |
Cyclopent-3-en-1-yl tosylate | 0.002 | 80% Ethanol/Water |
Tetramethylthis compound | 0.88 | Weakly Nucleophilic Media |
This data indicates that steric hindrance and electronic effects play crucial roles in determining reaction rates.
Q & A
Basic Research Questions
Q. What established methods are used to synthesize cyclopentyl tosylate, and how can reaction conditions be optimized for higher yields?
this compound is typically synthesized via the reaction of cyclopentanol with tosyl chloride in the presence of a base (e.g., pyridine). A published procedure achieved a 55% yield using this method . To optimize yields, researchers should systematically vary parameters such as reaction temperature (e.g., 0–25°C), stoichiometry of tosyl chloride, and solvent choice (e.g., dichloromethane vs. THF). Monitoring reaction progress via thin-layer chromatography (TLC) or gas chromatography (GC) can help identify optimal termination points. Post-synthesis purification via recrystallization or column chromatography may further improve purity .
Q. Which spectroscopic techniques are recommended for characterizing this compound, and how should data interpretation be approached?
Key techniques include nuclear magnetic resonance (NMR) spectroscopy for structural confirmation (e.g., distinguishing tosyl group protons at δ 7.2–7.8 ppm and cyclopentyl protons at δ 1.5–2.0 ppm) and infrared (IR) spectroscopy to confirm sulfonate ester formation (S=O stretching at ~1360 and 1170 cm⁻¹). Gas chromatography (GC) with flame ionization detection can assess purity, while mass spectrometry (MS) verifies molecular ion peaks (e.g., m/z 260 for C₁₂H₁₆O₃S) .
Q. What safety protocols are essential when handling this compound in laboratory settings?
this compound should be handled in a fume hood with nitrile gloves and lab coats. Avoid skin contact, as sulfonate esters can act as alkylating agents. Waste should be neutralized with a mild base (e.g., sodium bicarbonate) before disposal. While specific safety data for this compound are limited, general protocols for sulfonate esters apply: store in airtight containers at 2–8°C and avoid incompatible reagents (e.g., strong oxidizers) .
Advanced Research Questions
Q. How does the steric environment of this compound influence its reactivity in nucleophilic substitution compared to other cycloalkyl tosylates?
this compound’s reactivity is modulated by ring strain and steric hindrance. Cyclopentane’s envelope conformation reduces steric hindrance compared to cyclohexane’s chair form, enabling faster nucleophilic substitution. For example, this compound is reduced to cyclopentane in 15 minutes with LiAlH₄, whereas cyclohexyl tosylate requires 12 hours due to increased steric resistance . Computational studies (e.g., DFT calculations) can quantify transition-state energies, while kinetic experiments (e.g., rate comparisons under identical conditions) validate steric effects .
Q. What methodological approaches effectively analyze the kinetic profile of this compound reduction reactions?
Pseudo-first-order kinetics can be studied by monitoring reactant depletion via GC or NMR. For example, in LiAlH₄-mediated reductions, aliquots are quenched at intervals, and the residual tosylate is quantified. Activation parameters (ΔH‡, ΔS‡) are derived from Arrhenius plots across temperatures (e.g., 25–60°C). Isotopic labeling (e.g., deuterated solvents) may elucidate mechanistic pathways (e.g., SN2 vs. radical mechanisms) .
Q. How can computational modeling predict this compound’s reactivity in novel synthetic pathways?
Molecular dynamics (MD) simulations and quantum mechanical calculations (e.g., Hartree-Fock, MP2) model transition states and intermediates. For instance, docking studies can predict interactions with enzymes in catalytic applications. Validation involves comparing computed activation energies with experimental kinetic data. Tools like Gaussian or ORCA are standard for such analyses .
Q. How do data inconsistencies in this compound’s reaction outcomes arise, and what strategies resolve them?
Contradictions may stem from impurities, solvent effects, or unaccounted variables (e.g., moisture). Replicating experiments under controlled conditions (e.g., inert atmosphere, anhydrous solvents) and characterizing intermediates (e.g., by NMR) can identify confounding factors. Meta-analyses of published data (e.g., comparing yields across solvent systems) may reveal trends obscured in individual studies .
Q. Methodological Frameworks
Q. How can the PICOT framework structure research questions on this compound’s applications?
Adapt the PICOT (Population, Intervention, Comparison, Outcome, Time) model:
- Population : Reaction systems (e.g., cross-coupling reactions).
- Intervention : Use of this compound as a substrate.
- Comparison : Alternative leaving groups (e.g., mesylates, triflates).
- Outcome : Reaction yield, enantioselectivity, or rate constants.
- Time : Reaction duration or stability under storage. Example: “Does this compound (I) provide higher yields than mesylates (C) in Suzuki-Miyaura couplings (O) under ambient conditions (T)?” .
Q. What strategies ensure reproducibility in this compound studies?
Document all variables: reagent grades, equipment calibration (e.g., GC column type), and environmental conditions (humidity, temperature). Share raw data (e.g., NMR spectra) in supplementary materials. Use standardized protocols, such as those from The Journal of Organic Chemistry, and validate findings with independent replicates .
Q. Data Presentation and Analysis
Q. How should researchers present this compound data in publications to enhance clarity?
Use tables to compare reaction conditions (e.g., solvent, catalyst) and outcomes (yield, purity). Figures should highlight trends (e.g., kinetic plots, stereochemical outcomes). For example, a table contrasting reduction rates of cycloalkyl tosylates (cyclopentyl vs. cyclohexyl) with footnotes explaining anomalies (e.g., side-product formation) improves interpretability .
Properties
IUPAC Name |
cyclopentyl 4-methylbenzenesulfonate | |
---|---|---|
Source | PubChem | |
URL | https://pubchem.ncbi.nlm.nih.gov | |
Description | Data deposited in or computed by PubChem | |
InChI |
InChI=1S/C12H16O3S/c1-10-6-8-12(9-7-10)16(13,14)15-11-4-2-3-5-11/h6-9,11H,2-5H2,1H3 | |
Source | PubChem | |
URL | https://pubchem.ncbi.nlm.nih.gov | |
Description | Data deposited in or computed by PubChem | |
InChI Key |
ZWOQVPFVARFZSF-UHFFFAOYSA-N | |
Source | PubChem | |
URL | https://pubchem.ncbi.nlm.nih.gov | |
Description | Data deposited in or computed by PubChem | |
Canonical SMILES |
CC1=CC=C(C=C1)S(=O)(=O)OC2CCCC2 | |
Source | PubChem | |
URL | https://pubchem.ncbi.nlm.nih.gov | |
Description | Data deposited in or computed by PubChem | |
Molecular Formula |
C12H16O3S | |
Source | PubChem | |
URL | https://pubchem.ncbi.nlm.nih.gov | |
Description | Data deposited in or computed by PubChem | |
DSSTOX Substance ID |
DTXSID20312172 | |
Record name | cyclopentyl tosylate | |
Source | EPA DSSTox | |
URL | https://comptox.epa.gov/dashboard/DTXSID20312172 | |
Description | DSSTox provides a high quality public chemistry resource for supporting improved predictive toxicology. | |
Molecular Weight |
240.32 g/mol | |
Source | PubChem | |
URL | https://pubchem.ncbi.nlm.nih.gov | |
Description | Data deposited in or computed by PubChem | |
CAS No. |
3558-06-3 | |
Record name | NSC250985 | |
Source | DTP/NCI | |
URL | https://dtp.cancer.gov/dtpstandard/servlet/dwindex?searchtype=NSC&outputformat=html&searchlist=250985 | |
Description | The NCI Development Therapeutics Program (DTP) provides services and resources to the academic and private-sector research communities worldwide to facilitate the discovery and development of new cancer therapeutic agents. | |
Explanation | Unless otherwise indicated, all text within NCI products is free of copyright and may be reused without our permission. Credit the National Cancer Institute as the source. | |
Record name | cyclopentyl tosylate | |
Source | EPA DSSTox | |
URL | https://comptox.epa.gov/dashboard/DTXSID20312172 | |
Description | DSSTox provides a high quality public chemistry resource for supporting improved predictive toxicology. | |
Synthesis routes and methods I
Procedure details
Synthesis routes and methods II
Procedure details
Retrosynthesis Analysis
AI-Powered Synthesis Planning: Our tool employs the Template_relevance Pistachio, Template_relevance Bkms_metabolic, Template_relevance Pistachio_ringbreaker, Template_relevance Reaxys, Template_relevance Reaxys_biocatalysis model, leveraging a vast database of chemical reactions to predict feasible synthetic routes.
One-Step Synthesis Focus: Specifically designed for one-step synthesis, it provides concise and direct routes for your target compounds, streamlining the synthesis process.
Accurate Predictions: Utilizing the extensive PISTACHIO, BKMS_METABOLIC, PISTACHIO_RINGBREAKER, REAXYS, REAXYS_BIOCATALYSIS database, our tool offers high-accuracy predictions, reflecting the latest in chemical research and data.
Strategy Settings
Precursor scoring | Relevance Heuristic |
---|---|
Min. plausibility | 0.01 |
Model | Template_relevance |
Template Set | Pistachio/Bkms_metabolic/Pistachio_ringbreaker/Reaxys/Reaxys_biocatalysis |
Top-N result to add to graph | 6 |
Feasible Synthetic Routes
Disclaimer and Information on In-Vitro Research Products
Please be aware that all articles and product information presented on BenchChem are intended solely for informational purposes. The products available for purchase on BenchChem are specifically designed for in-vitro studies, which are conducted outside of living organisms. In-vitro studies, derived from the Latin term "in glass," involve experiments performed in controlled laboratory settings using cells or tissues. It is important to note that these products are not categorized as medicines or drugs, and they have not received approval from the FDA for the prevention, treatment, or cure of any medical condition, ailment, or disease. We must emphasize that any form of bodily introduction of these products into humans or animals is strictly prohibited by law. It is essential to adhere to these guidelines to ensure compliance with legal and ethical standards in research and experimentation.