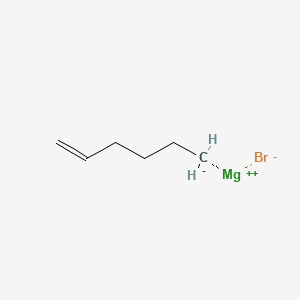
Hex-5-enylmagnesium bromide, 0.5M in THF
Overview
Description
Hex-5-enylmagnesium bromide, 0.5M in tetrahydrofuran (THF), is an organomagnesium compound commonly used in organic synthesis. It is a type of Grignard reagent, which is highly reactive and plays a crucial role in forming carbon-carbon bonds. The compound has the molecular formula C6H11BrMg and a molecular weight of 187.36 g/mol .
Preparation Methods
Hex-5-enylmagnesium bromide is typically prepared through the reaction of 5-bromo-1-hexene with magnesium metal in the presence of anhydrous THF as a solvent. The reaction is carried out under an inert atmosphere, usually nitrogen or argon, to prevent the highly reactive Grignard reagent from reacting with moisture or oxygen. The general reaction is as follows:
5-Bromo-1-hexene+Mg→Hex-5-enylmagnesium bromide
Chemical Reactions Analysis
Hex-5-enylmagnesium bromide undergoes various types of chemical reactions, including:
Nucleophilic Addition: It reacts with carbonyl compounds (aldehydes, ketones, esters) to form alcohols.
Substitution Reactions: It can participate in nucleophilic substitution reactions with alkyl halides to form new carbon-carbon bonds.
Coupling Reactions: It is used in coupling reactions with halides to form larger organic molecules.
Common reagents and conditions for these reactions include the use of anhydrous solvents, low temperatures, and inert atmospheres to prevent side reactions. Major products formed from these reactions are typically alcohols, alkanes, and complex organic molecules .
Scientific Research Applications
Hex-5-enylmagnesium bromide is widely used in scientific research due to its versatility in organic synthesis. Some of its applications include:
Chemistry: It is used to synthesize complex organic molecules, including pharmaceuticals, agrochemicals, and polymers.
Biology: It is employed in the synthesis of biologically active compounds and natural products.
Medicine: It is used in the development of new drugs and therapeutic agents.
Industry: It is utilized in the production of fine chemicals and materials with specific properties.
Mechanism of Action
The mechanism by which Hex-5-enylmagnesium bromide exerts its effects involves the nucleophilic attack of the carbon-magnesium bond on electrophilic centers, such as carbonyl groups. This reaction forms a new carbon-carbon bond, which is a fundamental step in building complex organic structures. The molecular targets are typically carbonyl compounds, and the pathways involved include nucleophilic addition and substitution reactions .
Comparison with Similar Compounds
Hex-5-enylmagnesium bromide can be compared with other Grignard reagents, such as methylmagnesium bromide and phenylmagnesium bromide. While all these compounds share similar reactivity patterns, Hex-5-enylmagnesium bromide is unique due to its hexenyl group, which provides additional synthetic flexibility. Similar compounds include:
- Methylmagnesium bromide
- Phenylmagnesium bromide
- Ethylmagnesium bromide
Each of these reagents has its own specific applications and advantages, depending on the desired synthetic outcome .
Properties
IUPAC Name |
magnesium;hex-1-ene;bromide | |
---|---|---|
Source | PubChem | |
URL | https://pubchem.ncbi.nlm.nih.gov | |
Description | Data deposited in or computed by PubChem | |
InChI |
InChI=1S/C6H11.BrH.Mg/c1-3-5-6-4-2;;/h3H,1-2,4-6H2;1H;/q-1;;+2/p-1 | |
Source | PubChem | |
URL | https://pubchem.ncbi.nlm.nih.gov | |
Description | Data deposited in or computed by PubChem | |
InChI Key |
NGTQJLNKMOCOQA-UHFFFAOYSA-M | |
Source | PubChem | |
URL | https://pubchem.ncbi.nlm.nih.gov | |
Description | Data deposited in or computed by PubChem | |
Canonical SMILES |
[CH2-]CCCC=C.[Mg+2].[Br-] | |
Source | PubChem | |
URL | https://pubchem.ncbi.nlm.nih.gov | |
Description | Data deposited in or computed by PubChem | |
Molecular Formula |
C6H11BrMg | |
Source | PubChem | |
URL | https://pubchem.ncbi.nlm.nih.gov | |
Description | Data deposited in or computed by PubChem | |
Molecular Weight |
187.36 g/mol | |
Source | PubChem | |
URL | https://pubchem.ncbi.nlm.nih.gov | |
Description | Data deposited in or computed by PubChem | |
Retrosynthesis Analysis
AI-Powered Synthesis Planning: Our tool employs the Template_relevance Pistachio, Template_relevance Bkms_metabolic, Template_relevance Pistachio_ringbreaker, Template_relevance Reaxys, Template_relevance Reaxys_biocatalysis model, leveraging a vast database of chemical reactions to predict feasible synthetic routes.
One-Step Synthesis Focus: Specifically designed for one-step synthesis, it provides concise and direct routes for your target compounds, streamlining the synthesis process.
Accurate Predictions: Utilizing the extensive PISTACHIO, BKMS_METABOLIC, PISTACHIO_RINGBREAKER, REAXYS, REAXYS_BIOCATALYSIS database, our tool offers high-accuracy predictions, reflecting the latest in chemical research and data.
Strategy Settings
Precursor scoring | Relevance Heuristic |
---|---|
Min. plausibility | 0.01 |
Model | Template_relevance |
Template Set | Pistachio/Bkms_metabolic/Pistachio_ringbreaker/Reaxys/Reaxys_biocatalysis |
Top-N result to add to graph | 6 |
Feasible Synthetic Routes
Disclaimer and Information on In-Vitro Research Products
Please be aware that all articles and product information presented on BenchChem are intended solely for informational purposes. The products available for purchase on BenchChem are specifically designed for in-vitro studies, which are conducted outside of living organisms. In-vitro studies, derived from the Latin term "in glass," involve experiments performed in controlled laboratory settings using cells or tissues. It is important to note that these products are not categorized as medicines or drugs, and they have not received approval from the FDA for the prevention, treatment, or cure of any medical condition, ailment, or disease. We must emphasize that any form of bodily introduction of these products into humans or animals is strictly prohibited by law. It is essential to adhere to these guidelines to ensure compliance with legal and ethical standards in research and experimentation.