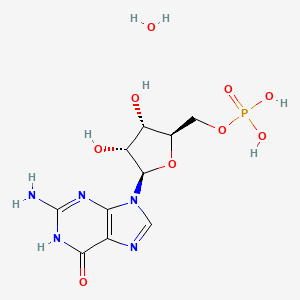
Guanosine 5'-monophosphate hydrate
Overview
Description
Guanosine 5'-monophosphate disodium salt hydrate (GMP, C₁₀H₁₂N₅Na₂O₈P) is a nucleotide derivative composed of a guanine base, ribose sugar, and a phosphate group at the 5'-position. It exists as a hydrate with two sodium counterions, giving it high solubility in aqueous solutions (>50 g/L) . GMP is naturally present in RNA and serves as a precursor in purine metabolism. Industrially, it is widely used as a flavor enhancer in foods due to its synergistic umami effect with inosine 5'-monophosphate (IMP) . GMP is also employed as a reference material in analytical chemistry and biochemical research, often derived from yeast fermentation .
Preparation Methods
Enzymatic Hydrolysis of Ribonucleic Acid (RNA)
RNA Extraction and Pretreatment
Ribonucleic acid (RNA) from yeast or microbial sources serves as the primary substrate. RNA is extracted via alkaline lysis, followed by precipitation with ethanol or isopropanol. The purified RNA is dissolved in a buffer (pH 5.0–6.0) and heated to 70°C for 30 minutes to denature secondary structures.
5′-Phosphodiesterase (5′-PDE)-Catalyzed Hydrolysis
5′-Phosphodiesterase hydrolyzes RNA into a mixture of 5′-nucleotides, including guanosine 5′-monophosphate (5′-GMP). Optimal conditions include:
The reaction yields 5′-GMP alongside cytidine, uridine, and adenosine monophosphates. Reverse-phase HPLC analysis quantifies 5′-GMP using a C-18 column with triethylammonium acetate (0.1 M, pH 8.8) and acetonitrile (99:1 v/v) as the mobile phase.
Key Data:
Parameter | Value |
---|---|
Hydrolysis yield | 15–20% (total nucleotides) |
5′-GMP purity | ≥95% after ion-exchange |
Microbial Fermentation
Two-Step Fermentation Using Bacillus subtilis
This industrial method involves two stages:
- Guanosine Production :
- Phosphorylation to 5′-GMP :
Key Data:
Parameter | Value |
---|---|
Fermentation broth yield | 570 µg/mL 5′-GMP |
Phosphorylation efficiency | >90% |
Biosynthesis-Chemical Synthesis Hybrid
- Step 1 : Bacillus megaterium ferments glucose (8%) at pH 7 for 90 hours, producing 15 g/L 5-amino-4-carboxamide nucleoside (AICAr).
- Step 2 : AICAr is converted to guanosine via thioglycoside intermediate formation (using CS$$2$$) and oxidation (H$$2$$O$$_2$$).
- Step 3 : Guanosine is phosphorylated using POCl$$_3$$.
Enzymatic Transphosphatidylation and Hydrolysis
Phospholipase D (PLD)-Catalyzed Transphosphatidylation
- Substrate : Phosphatidylcholine + guanosine.
- Enzyme : PLD from Streptomyces netropsis.
- Conditions : 37°C, 200 rpm, 24 hours.
- Product : 5′-(3-sn-phosphatidyl)guanosine.
Phospholipase C (PLC)-Catalyzed Hydrolysis
- Enzyme : PLC from Bacillus cereus.
- Conditions : 37°C, 100 mM HEPES buffer (pH 7.5), 50 mM CaCl$$_2$$.
- Yield : 75% 5′-GMP after 140 hours.
Crystallization and Hydrate Formation
Antisolvent Crystallization
Supersaturation-controlled crystallization selectively produces hydrate forms:
- Solvent : Water-ethanol mixtures.
- Variables : Temperature (10–40°C), initial concentration (0.1–0.4 g/mL), antisolvent addition rate.
Phase Transformation Stages:
- Nucleation of amorphous form.
- Predissolution of amorphous particles.
- Nucleation and growth of hydrate crystals.
- Dissolution of residual amorphous solid.
Hydrate Stability
Solubility Data:
Form | Solubility (g/100 mL H$$_2$$O) |
---|---|
Amorphous | 12.3 |
Heptahydrate | 8.7 |
Tetrahydrate | 6.9 |
Industrial Purification Techniques
Ion-Exchange Chromatography
Crude 5′-GMP is purified using Dowex-1-X2 resin:
- Eluent : 0.005 M HCl (removes guanine/guanosine), followed by 0.1 M HCl (elutes 5′-GMP).
- Recovery : >90%.
Crystallization Optimization
- Additives : NaCl (0.1–0.5 M) suppresses amorphous phase formation.
- Yield : 63% after calcium chloride precipitation.
Comparative Analysis of Methods
Method | Yield (%) | Purity (%) | Scalability | Cost |
---|---|---|---|---|
Enzymatic hydrolysis | 15–20 | 95 | Moderate | Low |
Microbial fermentation | 50–63 | 98 | High | Medium |
Enzymatic transphosphatidylation | 75 | 97 | Low | High |
Challenges and Innovations
- Nucleotidase Contamination : Bacillus subtilis strains require low nucleotidase activity to prevent 5′-GMP degradation.
- Crystallization Control : In situ Raman spectroscopy monitors phase transitions during antisolvent crystallization.
- Sustainable Processes : Agro-industrial wastes (e.g., soybean meal) reduce fermentation costs.
Chemical Reactions Analysis
Acid-Base Behavior and Deprotonation
GMP undergoes deprotonation at both the guanine amine (N1) and phosphate group under alkaline conditions, forming a dianionic species, . This dianion interacts with water molecules via hydrogen bonding, as shown by photoelectron spectroscopy and molecular dynamics simulations:
-
Microsolvation : Hydration with 1–4 water molecules increases adiabatic detachment energy (ADE) by 0.5–0.1 eV, stabilizing the dianion .
-
Proton Transfer : Clusters with ≥3 water molecules exhibit proton transfer between water and the phosphate group, modulating electronic properties .
Coordination with Metal Ions
GMP’s phosphate and nucleobase groups serve as binding sites for metal ions, influencing aggregation and structural stability:
Silver Ion (Ag⁺) Binding
Ag⁺ promotes GMP stacking and aggregation via:
-
Phosphate Interaction : Electrostatic binding to the dianionic phosphate .
-
N7 Coordination : Direct interaction with guanine’s N7 atom, confirmed by calorimetry and 7-deazaguanosine comparisons .
Ligand | ΔH (kcal/mol) | Binding Affinity (K, M⁻¹) | Stoichiometry (Ag⁺:Ligand) |
---|---|---|---|
5’-GMP | -9.8 ± 0.5 | 9.6 × 10⁴ | 2.3 ± 0.1 |
Guanosine | -14.7 ± 1.0 | 1.8 × 10⁵ | 1.33 ± 0.05 |
5’-CMP | -3.0 ± 0.8 | 1.3 × 10³ | 1.1 ± 0.3 |
Table 1: Thermodynamic parameters for Ag⁺ binding to GMP and analogs .
Alkali Metal Ion Effects
-
Na⁺/K⁺-Driven Self-Assembly : At pH 5, GMP forms a right-handed helix with a central channel devoid of Na⁺ . At pH 8, Na⁺ occupies the channel, stabilizing a left-handed structure .
-
Quadruplex Formation : K⁺ induces GMP aggregation into G-quadruplexes, while Na⁺ favors helical structures .
pH-Dependent Structural Transitions
GMP’s conformation and aggregation state are pH-sensitive:
-
pH 5 : Forms a right-handed helical gel with lock-washer-like guanine tetramers. The phosphate groups hydrogen-bond to guanine’s exocyclic amine (N2-H⋯O=P, distance ≈2.82 Å) .
-
pH 8 : Adopts a left-handed helix with stacked G-quartets and Na⁺ in the central channel .
Key Observations :
-
Solid-state ³¹P NMR shows a single peak at 1.3 ppm for pH 5 gels, indicating equivalent phosphate environments .
-
IR spectroscopy confirms C3′-endo sugar puckering in the acidic helix .
Self-Assembly and Aggregation
GMP’s aggregation is driven by π-π stacking, hydrogen bonding, and ion coordination:
-
Thermodynamics : Ag⁺-mediated aggregation is exothermic (ΔH = -9.8 kcal/mol) and entropy-driven at high ionic strength .
-
Structural Models :
Enzymatic and Synthetic Pathways
Scientific Research Applications
Biochemical Applications
1.1 Cell Biology and Culture
GMP is frequently used in cell culture systems to enhance cell growth and viability. It serves as a source of guanine nucleotides, which are essential for nucleic acid synthesis and cellular metabolism. Studies have shown that GMP can improve the proliferation of certain cell types, making it valuable in research involving cell lines.
1.2 Gene Expression and Transfection
GMP is utilized in transfection protocols to facilitate the introduction of nucleic acids into cells. Its role as a nucleotide helps in stabilizing RNA molecules, thus enhancing gene expression studies. This application is particularly significant in the development of gene therapies and vaccine production.
Neuroprotective Properties
Recent research has highlighted the neuroprotective effects of guanosine, particularly in neuropathologies such as ischemic stroke. Guanosine promotes neuronal survival and differentiation, making it a candidate for therapeutic strategies aimed at neuroprotection and recovery after CNS injuries .
Case Study: Guanosine's Effect on Neuronal Differentiation
A study demonstrated that guanosine could induce neurite outgrowth in neuroblastoma cells comparable to established differentiation agents like retinoic acid. This suggests its potential utility in therapies targeting neurodegenerative diseases .
Pharmaceutical Formulations
GMP is incorporated into various pharmaceutical formulations due to its biological activity and safety profile. It is often used as an excipient or active ingredient in medications aimed at enhancing cellular function or providing neuroprotection.
Analytical Chemistry
In analytical chemistry, GMP is employed as a standard for the quantification of nucleotides in biological samples. Its presence can indicate metabolic states or cellular responses to treatments, making it a valuable biomarker in research settings.
Food Industry Applications
GMP is also used as a flavor enhancer in the food industry, particularly within processed foods. Its ability to enhance umami flavors makes it popular in various culinary applications.
Research on Crystallization Processes
Research has focused on the crystallization behavior of GMP under different conditions, including the transformation from amorphous to crystalline forms . Understanding these processes is essential for optimizing GMP's use in various applications.
Mechanism of Action
The mechanism by which Guanosine 5'-monophosphate hydrate exerts its effects involves its role as a precursor for the synthesis of RNA. It is incorporated into RNA molecules during transcription, where it pairs with cytosine to form the G-C base pair. This base pairing is crucial for the stability and function of RNA molecules. Additionally, this compound is involved in signal transduction pathways, where it acts as a secondary messenger to transmit signals within cells.
Comparison with Similar Compounds
Comparison with Similar Nucleotides
Structural and Chemical Properties
GMP belongs to the family of 5'-monophosphate nucleotides, which include adenosine 5'-monophosphate (AMP), cytidine 5'-monophosphate (CMP), uridine 5'-monophosphate (UMP), and inosine 5'-monophosphate (IMP). Key differences lie in their nucleobases and resulting physicochemical properties:
Key Observations:
- Nucleobase Differences: GMP’s guanine base contains an exocyclic amine and carbonyl group, contributing to its distinct hydrogen-bonding capacity compared to adenine (AMP) or uracil (UMP) .
- Solubility: All 5'-monophosphates exhibit high water solubility due to their ionic nature, but subtle variations arise from nucleobase hydrophobicity.
- Stability: GMP and IMP are more heat-stable than cyclic nucleotides (e.g., cAMP), making them suitable for food processing .
Research Findings and Data
Taste Enhancement Studies
- In dried mushrooms, microwave vacuum drying (MVD) increased GMP content by 42% compared to fresh samples, yielding a umami equivalence (EUC) of 302.15 g MSG/100 g .
- Sensory panels rated GMP+IMP combinations 8× more potent than GMP alone .
Stability in Aqueous Solutions
- GMP remains stable in neutral buffers but degrades under acidic conditions (pH <3) due to glycosidic bond hydrolysis .
Biological Activity
Guanosine 5'-monophosphate (GMP) hydrate, a nucleotide and a key component in RNA synthesis, has garnered significant attention in the field of biological research due to its diverse biological activities. This article provides a comprehensive overview of GMP hydrate's biological activity, including its neuroprotective properties, role in cellular signaling, and potential therapeutic applications.
Structure and Synthesis
GMP is composed of a guanine base, a ribose sugar, and a phosphate group. It can exist in various hydrated forms, including the heptahydrate and tetrahydrate forms, which are produced through microbial fermentation processes . The synthesis of GMP begins with D-ribose 5'-phosphate, progressing through the de novo pathway involving multiple amino acids and one-carbon derivatives .
1. Neuroprotective Properties
GMP has been shown to exhibit neuroprotective effects, particularly in models of glutamate-induced neurotoxicity. In studies involving rat hippocampal slices, GMP administration was found to prevent neurotoxicity caused by glutamate during glucose deprivation conditions. This suggests that GMP may play a protective role in neurological conditions characterized by excitotoxicity .
2. Modulation of Glutamatergic Neurotransmission
Research indicates that GMP can modulate glutamatergic neurotransmission, which is crucial for synaptic plasticity and memory formation. The compound has been implicated in enhancing the survival and differentiation of neurons under stress conditions .
3. Induction of Neurite Outgrowth
Guanosine has been observed to promote neurite outgrowth in neuroblastoma cells, indicating its potential role in neuronal differentiation. This effect is comparable to that induced by known differentiation agents such as retinoic acid .
1. Formation of G-Quadruplexes
GMP self-aggregates in aqueous solutions to form G-quadruplex structures, which are important for various biological functions including telomerase activity regulation. The formation of these structures is influenced by ionic conditions and concentration of GMP .
2. Interaction with Nucleoside Transporters
GMP can cross the blood-brain barrier via nucleoside transporters, allowing it to exert its effects within the central nervous system shortly after administration. This rapid uptake highlights its potential for therapeutic use in neurological disorders .
Neuroprotection Against Ischemic Damage
A study demonstrated that systemic administration of GMP led to increased levels of guanosine in the cerebrospinal fluid following ischemic stroke models. The sustained elevation of guanosine levels post-injury suggests its role as an endogenous neuroprotective agent .
Anxiolytic Effects
Another significant finding is the anxiolytic-like behavior induced by GMP in animal models. This effect is attributed to its interaction with the glutamatergic system, making it a candidate for developing new anxiolytic therapies .
Research Findings Summary
Q & A
Basic Research Questions
Q. What methodologies are recommended for synthesizing and purifying GMP hydrate in laboratory settings?
- Methodology : Enzymatic synthesis using RNA hydrolysis (e.g., yeast-derived processes) followed by ion-exchange chromatography for purification. Monitor purity via HPLC with UV detection (λ = 254 nm) and validate using mass spectrometry (MS) or nuclear magnetic resonance (NMR) .
- Key Considerations : Ensure hydration stability by lyophilizing under controlled humidity (2-8°C storage recommended) .
Q. How can researchers confirm the structural integrity of GMP hydrate post-synthesis?
- Analytical Workflow :
Purity : Use reverse-phase HPLC with a C18 column (mobile phase: 10 mM ammonium acetate, pH 5.0) to separate GMP from impurities .
Hydration State : Thermogravimetric analysis (TGA) to quantify water content (4–8% typical for hydrates) .
Structural Confirmation : P NMR for phosphate group identification and H NMR for ribose proton characterization .
Q. What protocols ensure stability of GMP hydrate in aqueous solutions during experiments?
- Best Practices :
- Prepare fresh solutions in deionized water (solubility >50 g/L at 25°C) .
- Avoid repeated freeze-thaw cycles; store aliquots at -20°C with desiccants .
- Monitor pH-dependent degradation (stable at pH 6–8) using spectrophotometric assays .
Advanced Research Questions
Q. How can conflicting data on GMP hydrate’s binding affinity in nucleotide-protein interactions be resolved?
- Troubleshooting Strategy :
Control for Hydration Effects : Compare binding kinetics of anhydrous vs. hydrated forms using isothermal titration calorimetry (ITC) .
Counterion Impact : Assess sodium vs. other counterions (e.g., potassium) in buffer systems (e.g., Tris-HCl, pH 7.4) .
Data Normalization : Use internal standards (e.g., ATP disodium salt) to calibrate assays .
Q. What experimental designs are optimal for studying GMP hydrate’s role in glutamatergic neurotransmission?
- In Vitro Approach :
- Primary Neuronal Cultures : Treat with 10–100 µM GMP hydrate and measure glutamate release via fluorometric assays .
- Receptor Binding : Use radiolabeled H-GMP to quantify NMDA/AMPA receptor interactions in synaptic membranes .
- In Vivo Model : Microdialysis in rodent brains with LC-MS/MS detection .
Q. How do variations in GMP hydrate’s physical form (e.g., crystalline vs. amorphous) affect enzymatic assays?
- Experimental Design :
Crystallinity Analysis : X-ray diffraction (XRD) to confirm crystalline structure .
Activity Assays : Compare Michaelis-Menten kinetics of GMP-dependent enzymes (e.g., guanylate kinase) using crystalline vs. lyophilized forms .
Solubility Optimization : Use co-solvents (e.g., DMSO ≤1%) for amorphous forms in cell-free systems .
Q. Methodological Challenges & Solutions
Q. How to address discrepancies in thermodynamic data for GMP hydrate across studies?
- Root Causes :
- Hydration state inconsistencies (e.g., monohydrate vs. trihydrate) .
- Buffer ion interference (e.g., sodium vs. magnesium in ITC) .
Q. What strategies improve detection limits for GMP hydrate in complex biological matrices?
- Advanced Techniques :
- HPLC-MS/MS : Use a hydrophilic interaction liquid chromatography (HILIC) column with MRM transitions (m/z 362 → 152) .
- Enzymatic Amplification : Couple with NADH-linked assays (e.g., GMP reductase) for signal enhancement .
Properties
CAS No. |
26166-34-7 |
---|---|
Molecular Formula |
C10H20N5O11P |
Molecular Weight |
417.27 g/mol |
IUPAC Name |
[(2R,3S,4R,5R)-5-(2-amino-6-oxo-1H-purin-9-yl)-3,4-dihydroxyoxolan-2-yl]methyl dihydrogen phosphate;trihydrate |
InChI |
InChI=1S/C10H14N5O8P.3H2O/c11-10-13-7-4(8(18)14-10)12-2-15(7)9-6(17)5(16)3(23-9)1-22-24(19,20)21;;;/h2-3,5-6,9,16-17H,1H2,(H2,19,20,21)(H3,11,13,14,18);3*1H2/t3-,5-,6-,9-;;;/m1.../s1 |
InChI Key |
GDVRLXWOAKUVHM-CYCLDIHTSA-N |
SMILES |
C1=NC2=C(N1C3C(C(C(O3)COP(=O)(O)O)O)O)N=C(NC2=O)N.O |
Isomeric SMILES |
C1=NC2=C(N1[C@H]3[C@@H]([C@@H]([C@H](O3)COP(=O)(O)O)O)O)N=C(NC2=O)N.O.O.O |
Canonical SMILES |
C1=NC2=C(N1C3C(C(C(O3)COP(=O)(O)O)O)O)N=C(NC2=O)N.O.O.O |
Key on ui other cas no. |
85-32-5 |
sequence |
G |
Synonyms |
5' Guanylic Acid 5'-GMP 5'-Guanylic Acid 5'-Monophosphate, Guanosine Acid, 5'-Guanylic Acid, Guanylic Guanosine 5' Monophosphate Guanosine 5'-Monophosphate Guanosine Monophosphate Guanylic Acid Monophosphate, Guanosine |
Origin of Product |
United States |
Retrosynthesis Analysis
AI-Powered Synthesis Planning: Our tool employs the Template_relevance Pistachio, Template_relevance Bkms_metabolic, Template_relevance Pistachio_ringbreaker, Template_relevance Reaxys, Template_relevance Reaxys_biocatalysis model, leveraging a vast database of chemical reactions to predict feasible synthetic routes.
One-Step Synthesis Focus: Specifically designed for one-step synthesis, it provides concise and direct routes for your target compounds, streamlining the synthesis process.
Accurate Predictions: Utilizing the extensive PISTACHIO, BKMS_METABOLIC, PISTACHIO_RINGBREAKER, REAXYS, REAXYS_BIOCATALYSIS database, our tool offers high-accuracy predictions, reflecting the latest in chemical research and data.
Strategy Settings
Precursor scoring | Relevance Heuristic |
---|---|
Min. plausibility | 0.01 |
Model | Template_relevance |
Template Set | Pistachio/Bkms_metabolic/Pistachio_ringbreaker/Reaxys/Reaxys_biocatalysis |
Top-N result to add to graph | 6 |
Feasible Synthetic Routes
Disclaimer and Information on In-Vitro Research Products
Please be aware that all articles and product information presented on BenchChem are intended solely for informational purposes. The products available for purchase on BenchChem are specifically designed for in-vitro studies, which are conducted outside of living organisms. In-vitro studies, derived from the Latin term "in glass," involve experiments performed in controlled laboratory settings using cells or tissues. It is important to note that these products are not categorized as medicines or drugs, and they have not received approval from the FDA for the prevention, treatment, or cure of any medical condition, ailment, or disease. We must emphasize that any form of bodily introduction of these products into humans or animals is strictly prohibited by law. It is essential to adhere to these guidelines to ensure compliance with legal and ethical standards in research and experimentation.