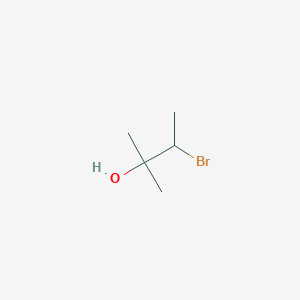
2-Methyl-3-bromo-2-butanol
Overview
Description
2-Methyl-3-bromo-2-butanol is an organic compound with the molecular formula C5H11BrO It is a brominated alcohol, characterized by the presence of a bromine atom and a hydroxyl group attached to a butane backbone
Preparation Methods
Synthetic Routes and Reaction Conditions: 2-Methyl-3-bromo-2-butanol can be synthesized through several methods. One common approach involves the bromination of 2-methyl-2-butanol using hydrogen bromide (HBr) or phosphorus tribromide (PBr3) under controlled conditions . Another method includes the addition of bromine to 2-methyl-2-butene, followed by hydrolysis to yield the desired alcohol .
Industrial Production Methods: In an industrial setting, the production of this compound typically involves large-scale bromination reactions. The use of continuous flow reactors can enhance the efficiency and yield of the process. The reaction conditions, such as temperature, pressure, and the concentration of reagents, are optimized to ensure high purity and minimal by-products.
Chemical Reactions Analysis
Types of Reactions: 2-Methyl-3-bromo-2-butanol undergoes various chemical reactions, including:
Substitution Reactions: The bromine atom can be replaced by other nucleophiles, such as hydroxide ions, to form different alcohols.
Elimination Reactions: Under basic conditions, the compound can undergo dehydrohalogenation to form alkenes.
Oxidation Reactions: The hydroxyl group can be oxidized to form ketones or aldehydes, depending on the reaction conditions.
Common Reagents and Conditions:
Substitution: Sodium hydroxide (NaOH) or potassium hydroxide (KOH) in aqueous or alcoholic solutions.
Elimination: Strong bases like sodium ethoxide (NaOEt) or potassium tert-butoxide (KOtBu).
Oxidation: Oxidizing agents such as potassium permanganate (KMnO4) or chromium trioxide (CrO3).
Major Products:
Substitution: Formation of different alcohols or ethers.
Elimination: Formation of alkenes like 2-methyl-2-butene.
Oxidation: Formation of ketones or aldehydes.
Scientific Research Applications
2-Methyl-3-bromo-2-butanol has several applications in scientific research:
Chemistry: Used as an intermediate in organic synthesis and as a reagent in various chemical reactions.
Biology: Employed in the study of enzyme-catalyzed reactions and metabolic pathways involving brominated compounds.
Medicine: Investigated for its potential use in the synthesis of pharmaceutical compounds and as a building block for drug development.
Mechanism of Action
The mechanism of action of 2-Methyl-3-bromo-2-butanol involves its reactivity as a brominated alcohol. The bromine atom and hydroxyl group confer unique chemical properties, allowing the compound to participate in various nucleophilic substitution and elimination reactions. The molecular targets and pathways involved depend on the specific reaction conditions and the nature of the reactants.
Comparison with Similar Compounds
2-Methyl-2-butanol: A non-brominated analog with similar structural features but different reactivity.
3-Bromo-2-methyl-2-butene: A brominated alkene with distinct chemical properties compared to the alcohol.
2-Bromo-3-methylbutane: Another brominated compound with a different substitution pattern on the butane backbone.
Uniqueness: 2-Methyl-3-bromo-2-butanol is unique due to the presence of both a bromine atom and a hydroxyl group on the same carbon atom. This dual functionality allows it to undergo a wide range of chemical reactions, making it a versatile compound in organic synthesis and industrial applications.
Properties
IUPAC Name |
3-bromo-2-methylbutan-2-ol | |
---|---|---|
Source | PubChem | |
URL | https://pubchem.ncbi.nlm.nih.gov | |
Description | Data deposited in or computed by PubChem | |
InChI |
InChI=1S/C5H11BrO/c1-4(6)5(2,3)7/h4,7H,1-3H3 | |
Source | PubChem | |
URL | https://pubchem.ncbi.nlm.nih.gov | |
Description | Data deposited in or computed by PubChem | |
InChI Key |
YGHWXEJVGBPVSX-UHFFFAOYSA-N | |
Source | PubChem | |
URL | https://pubchem.ncbi.nlm.nih.gov | |
Description | Data deposited in or computed by PubChem | |
Canonical SMILES |
CC(C(C)(C)O)Br | |
Source | PubChem | |
URL | https://pubchem.ncbi.nlm.nih.gov | |
Description | Data deposited in or computed by PubChem | |
Molecular Formula |
C5H11BrO | |
Source | PubChem | |
URL | https://pubchem.ncbi.nlm.nih.gov | |
Description | Data deposited in or computed by PubChem | |
DSSTOX Substance ID |
DTXSID10338285 | |
Record name | 3-Bromo-2-methyl-2-butanol | |
Source | EPA DSSTox | |
URL | https://comptox.epa.gov/dashboard/DTXSID10338285 | |
Description | DSSTox provides a high quality public chemistry resource for supporting improved predictive toxicology. | |
Molecular Weight |
167.04 g/mol | |
Source | PubChem | |
URL | https://pubchem.ncbi.nlm.nih.gov | |
Description | Data deposited in or computed by PubChem | |
CAS No. |
2588-77-4 | |
Record name | 3-Bromo-2-methyl-2-butanol | |
Source | EPA DSSTox | |
URL | https://comptox.epa.gov/dashboard/DTXSID10338285 | |
Description | DSSTox provides a high quality public chemistry resource for supporting improved predictive toxicology. | |
Retrosynthesis Analysis
AI-Powered Synthesis Planning: Our tool employs the Template_relevance Pistachio, Template_relevance Bkms_metabolic, Template_relevance Pistachio_ringbreaker, Template_relevance Reaxys, Template_relevance Reaxys_biocatalysis model, leveraging a vast database of chemical reactions to predict feasible synthetic routes.
One-Step Synthesis Focus: Specifically designed for one-step synthesis, it provides concise and direct routes for your target compounds, streamlining the synthesis process.
Accurate Predictions: Utilizing the extensive PISTACHIO, BKMS_METABOLIC, PISTACHIO_RINGBREAKER, REAXYS, REAXYS_BIOCATALYSIS database, our tool offers high-accuracy predictions, reflecting the latest in chemical research and data.
Strategy Settings
Precursor scoring | Relevance Heuristic |
---|---|
Min. plausibility | 0.01 |
Model | Template_relevance |
Template Set | Pistachio/Bkms_metabolic/Pistachio_ringbreaker/Reaxys/Reaxys_biocatalysis |
Top-N result to add to graph | 6 |
Feasible Synthetic Routes
Disclaimer and Information on In-Vitro Research Products
Please be aware that all articles and product information presented on BenchChem are intended solely for informational purposes. The products available for purchase on BenchChem are specifically designed for in-vitro studies, which are conducted outside of living organisms. In-vitro studies, derived from the Latin term "in glass," involve experiments performed in controlled laboratory settings using cells or tissues. It is important to note that these products are not categorized as medicines or drugs, and they have not received approval from the FDA for the prevention, treatment, or cure of any medical condition, ailment, or disease. We must emphasize that any form of bodily introduction of these products into humans or animals is strictly prohibited by law. It is essential to adhere to these guidelines to ensure compliance with legal and ethical standards in research and experimentation.