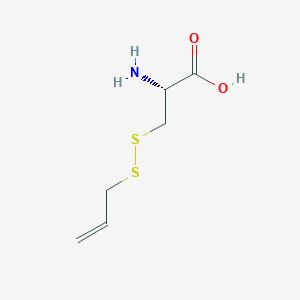
s-Allylmercaptocysteine
Overview
Description
S-Allylmercaptocysteine (SAMC) is a water-soluble organosulfur compound derived from aged garlic extract (AGE), formed via enzymatic and non-enzymatic transformations during garlic aging . Its chemical structure (CH₂=CH-CH₂-S-S-CH₂-CH-NH₂-COOH) includes a disulfide bond and a cysteine moiety, contributing to its antioxidant, anti-inflammatory, and antiproliferative properties . SAMC scavenges reactive oxygen species (ROS) and reactive nitrogen species (RNS), enhances antioxidant enzyme activity (e.g., glutathione peroxidase, catalase), and mitigates oxidative stress in renal, hepatic, and cardiovascular systems . Notably, SAMC exhibits chemopreventive effects in colon, lung, and other cancers by inducing apoptosis, cell cycle arrest, and immune modulation .
Preparation Methods
Synthetic Routes and Reaction Conditions: S-Allylmercaptocysteine can be synthesized through the reaction of allyl bromide with L-cysteine in the presence of a base such as sodium hydroxide . The reaction typically occurs in an aqueous medium at room temperature, resulting in the formation of this compound.
Industrial Production Methods: Industrial production of this compound involves the extraction of aged garlic, followed by purification processes to isolate the compound . The extraction process includes soaking raw garlic in aqueous ethanol for several months, during which the odorous and irritating constituents are transformed into non-toxic compounds .
Chemical Reactions Analysis
Types of Reactions: S-Allylmercaptocysteine undergoes various chemical reactions, including oxidation, reduction, and substitution .
Common Reagents and Conditions:
Reduction: Reduction of this compound can be achieved using reducing agents such as sodium borohydride.
Major Products Formed: The major products formed from these reactions include sulfoxides, sulfones, and various substituted derivatives .
Scientific Research Applications
Hepatoprotection
Mechanisms and Efficacy:
SAMC has demonstrated significant hepatoprotective effects against various liver diseases, including acute liver injury, non-alcoholic fatty liver disease (NAFLD), and liver cancer. Research indicates that SAMC can mitigate oxidative stress and inflammation in liver tissues.
-
Case Study: Alcoholic Liver Disease
A study highlighted SAMC's ability to protect against ethanol-induced liver injury. The compound restored insulin signaling pathways in hepatocytes, suggesting a mechanism involving the binding to insulin receptors and subsequent activation of downstream signaling pathways such as IRS-1/AKT/GSK3 . - Data Table: Hepatoprotective Effects of SAMC
Cancer Prevention
Anti-Cancer Properties:
SAMC exhibits potential anti-cancer properties across various malignancies, including gastric, colon, prostate, ovarian, and thyroid cancers. Its mechanisms include modulation of oncogenic pathways and inhibition of cancer cell proliferation.
-
Case Study: Prostate Cancer
Research has shown that SAMC can alter the expression of androgen-responsive biomarkers, indicating its role in prostate carcinogenesis prevention . It operates through pathways that inhibit fatty acid metabolism and enhance cytotoxicity against cancer cells. - Data Table: Anti-Cancer Effects of SAMC
Immunomodulation
Immune System Enhancement:
SAMC has been studied for its immunomodulatory effects, particularly in enhancing the immune response while mitigating excessive inflammation.
-
Case Study: Acute Respiratory Distress Syndrome (ARDS)
A patent describes a method utilizing SAMC to enhance immune responses against infections while reducing toxicity associated with immune reactions . This dual action makes SAMC a candidate for preventing and treating infectious diseases. - Data Table: Immunomodulatory Effects of SAMC
Mechanism of Action
S-Allylmercaptocysteine exerts its effects through various molecular targets and pathways. It induces apoptosis in cancer cells by activating the TGF-β signaling pathway and reducing NF-κB activity . Additionally, it upregulates Nrf2, leading to enhanced antioxidant responses. The compound also interacts with calpain, a calcium-dependent cysteine protease, to exert neuroprotective effects.
Comparison with Similar Compounds
S-Allylcysteine (SAC)
Mechanistic Differences : SAMC’s disulfide bond enhances thiol-mediated redox regulation, while SAC’s cysteine-derived structure supports glutathione synthesis .
Allicin
Structural Impact : Allicin’s thiosulfinate group confers rapid reactivity, whereas SAMC’s stability enables prolonged bioactivity .
Diallyl Disulfide (DADS) and Allylmercaptan (AM)
Functional Contrast : AM’s sulfhydryl group enhances HDAC inhibition, while SAMC’s disulfide bond supports immune-mediated antitumor effects .
Diallyl Trisulfide (DATS) and Ajoene
Key Insight : Lipid-soluble sulfides (e.g., DATS) excel in membrane permeability, while SAMC’s solubility favors systemic antioxidant effects .
Research Findings and Clinical Implications
- Renal Protection : SAMC (20 mg/kg) reduced gentamicin-induced oxidative stress by 47% (CAT activity) and 44% (GPx activity), outperforming SAC in renal enzyme restoration .
- Colon Cancer : SAMC (50 μM) induced 60% apoptosis in HT-29 cells via caspase-3 activation, contrasting SAC’s minimal effect .
- Immune Modulation : SAMC suppressed PD-L1 expression by 40% in murine models, enhancing T-cell-mediated tumor clearance .
- Bioavailability : SAMC content in AGE peaks at 4 months (2.1 mg/g), ensuring sustained release compared to transient allicin .
Biological Activity
S-Allylmercaptocysteine (SAMC) is a sulfur-containing compound derived from garlic, known for its various biological activities, particularly in cancer prevention and treatment. This article explores the biological activity of SAMC, focusing on its antiproliferative effects, mechanisms of action, and potential therapeutic applications.
Antiproliferative Effects
SAMC has been extensively studied for its ability to inhibit cell proliferation in various cancer cell lines. Notably, research has demonstrated that SAMC exhibits significant antiproliferative activity against erythroleukemia cells. In a study involving two erythroleukemia cell lines, HEL and OCIM-1, SAMC induced a dose-dependent inhibition of cell growth with 50% lethal doses of 0.046 mM and 0.093 mM, respectively .
Table 1: Antiproliferative Activity of SAMC
Cell Line | 50% Lethal Dose (mM) | Mechanism of Action |
---|---|---|
HEL | 0.093 | Induction of apoptosis |
OCIM-1 | 0.046 | Induction of apoptosis |
The mechanisms underlying the biological activity of SAMC are multifaceted. Key findings include:
- Induction of Apoptosis : SAMC triggers apoptosis in cancer cells through several pathways. For instance, in human colorectal carcinoma cells (SW620), SAMC was shown to activate the c-Jun N-terminal kinase (JNK) and p38 mitogen-activated protein kinase (p38) signaling pathways, leading to increased expression of pro-apoptotic factors such as p53 and Bax .
- Inhibition of Metastasis : In vivo studies have indicated that SAMC significantly reduces tumor growth and metastasis in models of androgen-independent prostate cancer. It achieved up to a 71% reduction in primary tumor growth and an 85.5% decrease in lung and adrenal metastases without notable toxicity .
Table 2: Effects of SAMC on Tumor Growth and Metastasis
Treatment Dose (mg/kg/d) | Tumor Growth Reduction (%) | Metastasis Reduction (%) |
---|---|---|
Control | 0 | 0 |
Low Dose (150) | Not significant | Not significant |
High Dose (300) | 71 | 85.5 |
Clinical Implications
The promising results from preclinical studies suggest that SAMC may serve as a novel therapeutic agent for various cancers. Its ability to modulate key signaling pathways involved in cell proliferation and apoptosis positions it as a potential candidate for clinical trials aimed at treating cancers such as prostate and colorectal carcinoma.
Case Studies
- Prostate Cancer : A study demonstrated that SAMC treatment resulted in significant suppression of tumor growth and metastasis in SCID mouse models of androgen-independent prostate cancer. High doses were well-tolerated with no observable toxicity, indicating its potential for safe clinical application .
- Colorectal Carcinoma : Another investigation revealed that SAMC reduced cell viability in SW620 cells through apoptosis induction via JNK and p38 pathways. The effective concentration for inducing apoptosis was determined to be 400 μM .
Q & A
Basic Research Questions
Q. What molecular mechanisms underlie SAMC's anticancer activity?
SAMC induces apoptosis in cancer cells through multiple pathways:
- TGF-β signaling : SAMC activates TGF-β-dependent apoptosis in ovarian cancer cells, particularly in cells with low survivin expression .
- ROS-mediated pathways : SAMC triggers G2/M phase arrest and apoptosis in colon cancer cells by increasing intracellular ROS, which activates p38 and JNK signaling .
- NF-κB and Nrf2 modulation : SAMC suppresses NF-κB (pro-inflammatory) and upregulates Nrf2 (antioxidant), reducing oxidative stress and inflammation . Methodological Insight: Use Western blotting to analyze TGF-β/Smad3 activation, flow cytometry for ROS quantification, and luciferase assays to measure NF-κB/Nrf2 transcriptional activity.
Q. How does SAMC mitigate oxidative stress in experimental models?
SAMC scavenges reactive oxygen species (ROS) and preserves antioxidant enzyme activity:
- Hydroxyl radical (•OH) and singlet oxygen (¹O₂) scavenging : Demonstrated in vitro using deoxyribose degradation and histidine photo-oxidation assays .
- Enzyme preservation : In gentamicin-induced nephrotoxicity, SAMC prevents declines in Mn-SOD, GPx, and GR activity but reduces catalase (CAT) activity, likely due to direct H₂O₂ scavenging . Methodological Insight: Quantify ROS using fluorescent probes (e.g., DCFH-DA) and measure enzyme activities via spectrophotometric assays (e.g., NBT for SOD, NADPH oxidation for GR) .
Advanced Research Questions
Q. How do experimental designs address conflicting data on SAMC's modulation of antioxidant enzymes?
Discrepancies in SAMC's effects on CAT activity (e.g., reduced in gentamicin models vs. no change in cisplatin models ) highlight context-dependent mechanisms:
- Dose and model specificity : SAMC’s H₂O₂-scavenging capacity may bypass CAT in high oxidative stress conditions (e.g., gentamicin), whereas in cisplatin models, Nrf2-mediated upregulation of endogenous antioxidants compensates .
- Temporal factors : CAT activity may rebound post-SAMC treatment due to adaptive Nrf2 signaling. Methodological Insight: Conduct time-course studies with SAMC and compare oxidative stress markers (e.g., 4-HNE, 3-NT) across models using immunohistochemistry .
Q. What in vivo models best elucidate SAMC's nephroprotective effects?
- Gentamicin-induced nephrotoxicity : SAMC reduces tubular damage by 38% and normalizes Mn-SOD/GPx activity, validated via urinary biomarkers (e.g., NAG, BUN) and histopathology .
- Cisplatin-induced nephrotoxicity : SAMC attenuates apoptosis by suppressing caspase-3 and Bax/Bcl-2 imbalance, confirmed via TUNEL staining and renal function tests (e.g., serum creatinine) . Methodological Insight: Use strain-specific rats (e.g., Sprague-Dawley) and standardize SAMC dosing (e.g., 50–100 mg/kg/day) to ensure reproducibility.
Q. How does survivin overexpression influence SAMC's efficacy in ovarian cancer?
Survivin, an anti-apoptotic protein, diminishes SAMC’s pro-apoptotic effects in ovarian cancer cells. Studies show:
- Survivin-overexpressing cells resist SAMC-induced apoptosis, necessitating combination therapies (e.g., SAMC + survivin inhibitors) .
- Mechanistic studies link survivin to SAMC’s failure to activate caspase-9 in resistant cells. Methodological Insight: Employ siRNA knockdown of survivin and assess apoptosis via Annexin V/PI staining .
Q. Methodological Considerations
Q. What assays are critical for evaluating SAMC's anti-inflammatory activity?
- NF-κB inhibition : Use electrophoretic mobility shift assays (EMSA) or p65 nuclear translocation imaging in macrophages stimulated with LPS .
- Cytokine profiling : Quantify TNF-α, IL-6, and IL-1β via ELISA in serum or tissue homogenates .
- Nrf2 activation : Measure NQO1 and HO-1 expression via qPCR or Western blotting in SAMC-treated cells .
Q. How can researchers reconcile SAMC's dual pro-oxidant (anticancer) and antioxidant (nephroprotective) roles?
- Context-dependent ROS modulation : Low SAMC doses act as antioxidants in normal cells by scavenging ROS, while higher doses induce ROS overload in cancer cells, triggering apoptosis .
- Cell-type specificity : Cancer cells (with impaired redox homeostasis) are more vulnerable to SAMC-induced ROS than normal cells. Methodological Insight: Compare ROS thresholds in cancer vs. normal cells using redox-sensitive dyes (e.g., DHE for superoxide) .
Q. Data Contradiction Analysis
Q. Why do some studies report SAMC-induced apoptosis via TGF-β, while others emphasize ROS?
- Cancer type variability : TGF-β signaling dominates in ovarian and gastric cancers, whereas ROS-driven apoptosis is prominent in colon and prostate cancers .
- Experimental conditions : Hypoxic vs. normoxic environments alter SAMC’s mechanistic emphasis. Methodological Insight: Use gene-editing tools (e.g., CRISPR for TGF-β receptors) to isolate pathway contributions .
Q. Tables for Key Findings
Properties
IUPAC Name |
(2R)-2-amino-3-(prop-2-enyldisulfanyl)propanoic acid | |
---|---|---|
Source | PubChem | |
URL | https://pubchem.ncbi.nlm.nih.gov | |
Description | Data deposited in or computed by PubChem | |
InChI |
InChI=1S/C6H11NO2S2/c1-2-3-10-11-4-5(7)6(8)9/h2,5H,1,3-4,7H2,(H,8,9)/t5-/m0/s1 | |
Source | PubChem | |
URL | https://pubchem.ncbi.nlm.nih.gov | |
Description | Data deposited in or computed by PubChem | |
InChI Key |
WYQZZUUUOXNSCS-YFKPBYRVSA-N | |
Source | PubChem | |
URL | https://pubchem.ncbi.nlm.nih.gov | |
Description | Data deposited in or computed by PubChem | |
Canonical SMILES |
C=CCSSCC(C(=O)O)N | |
Source | PubChem | |
URL | https://pubchem.ncbi.nlm.nih.gov | |
Description | Data deposited in or computed by PubChem | |
Isomeric SMILES |
C=CCSSC[C@@H](C(=O)O)N | |
Source | PubChem | |
URL | https://pubchem.ncbi.nlm.nih.gov | |
Description | Data deposited in or computed by PubChem | |
Molecular Formula |
C6H11NO2S2 | |
Source | PubChem | |
URL | https://pubchem.ncbi.nlm.nih.gov | |
Description | Data deposited in or computed by PubChem | |
DSSTOX Substance ID |
DTXSID401311848 | |
Record name | S-Allylmercapto-L-cysteine | |
Source | EPA DSSTox | |
URL | https://comptox.epa.gov/dashboard/DTXSID401311848 | |
Description | DSSTox provides a high quality public chemistry resource for supporting improved predictive toxicology. | |
Molecular Weight |
193.3 g/mol | |
Source | PubChem | |
URL | https://pubchem.ncbi.nlm.nih.gov | |
Description | Data deposited in or computed by PubChem | |
CAS No. |
2281-22-3 | |
Record name | S-Allylmercapto-L-cysteine | |
Source | CAS Common Chemistry | |
URL | https://commonchemistry.cas.org/detail?cas_rn=2281-22-3 | |
Description | CAS Common Chemistry is an open community resource for accessing chemical information. Nearly 500,000 chemical substances from CAS REGISTRY cover areas of community interest, including common and frequently regulated chemicals, and those relevant to high school and undergraduate chemistry classes. This chemical information, curated by our expert scientists, is provided in alignment with our mission as a division of the American Chemical Society. | |
Explanation | The data from CAS Common Chemistry is provided under a CC-BY-NC 4.0 license, unless otherwise stated. | |
Record name | S-Allylmercapto-L-cysteine | |
Source | EPA DSSTox | |
URL | https://comptox.epa.gov/dashboard/DTXSID401311848 | |
Description | DSSTox provides a high quality public chemistry resource for supporting improved predictive toxicology. | |
Record name | (2R)-2-amino-3-(prop-2-en-1-yldisulfanyl)propanoic acid | |
Source | European Chemicals Agency (ECHA) | |
URL | https://echa.europa.eu/information-on-chemicals | |
Description | The European Chemicals Agency (ECHA) is an agency of the European Union which is the driving force among regulatory authorities in implementing the EU's groundbreaking chemicals legislation for the benefit of human health and the environment as well as for innovation and competitiveness. | |
Explanation | Use of the information, documents and data from the ECHA website is subject to the terms and conditions of this Legal Notice, and subject to other binding limitations provided for under applicable law, the information, documents and data made available on the ECHA website may be reproduced, distributed and/or used, totally or in part, for non-commercial purposes provided that ECHA is acknowledged as the source: "Source: European Chemicals Agency, http://echa.europa.eu/". Such acknowledgement must be included in each copy of the material. ECHA permits and encourages organisations and individuals to create links to the ECHA website under the following cumulative conditions: Links can only be made to webpages that provide a link to the Legal Notice page. | |
Retrosynthesis Analysis
AI-Powered Synthesis Planning: Our tool employs the Template_relevance Pistachio, Template_relevance Bkms_metabolic, Template_relevance Pistachio_ringbreaker, Template_relevance Reaxys, Template_relevance Reaxys_biocatalysis model, leveraging a vast database of chemical reactions to predict feasible synthetic routes.
One-Step Synthesis Focus: Specifically designed for one-step synthesis, it provides concise and direct routes for your target compounds, streamlining the synthesis process.
Accurate Predictions: Utilizing the extensive PISTACHIO, BKMS_METABOLIC, PISTACHIO_RINGBREAKER, REAXYS, REAXYS_BIOCATALYSIS database, our tool offers high-accuracy predictions, reflecting the latest in chemical research and data.
Strategy Settings
Precursor scoring | Relevance Heuristic |
---|---|
Min. plausibility | 0.01 |
Model | Template_relevance |
Template Set | Pistachio/Bkms_metabolic/Pistachio_ringbreaker/Reaxys/Reaxys_biocatalysis |
Top-N result to add to graph | 6 |
Feasible Synthetic Routes
Disclaimer and Information on In-Vitro Research Products
Please be aware that all articles and product information presented on BenchChem are intended solely for informational purposes. The products available for purchase on BenchChem are specifically designed for in-vitro studies, which are conducted outside of living organisms. In-vitro studies, derived from the Latin term "in glass," involve experiments performed in controlled laboratory settings using cells or tissues. It is important to note that these products are not categorized as medicines or drugs, and they have not received approval from the FDA for the prevention, treatment, or cure of any medical condition, ailment, or disease. We must emphasize that any form of bodily introduction of these products into humans or animals is strictly prohibited by law. It is essential to adhere to these guidelines to ensure compliance with legal and ethical standards in research and experimentation.