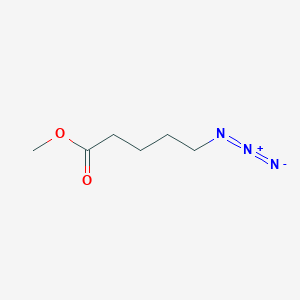
Methyl 5-azidopentanoate
Overview
Description
Preparation Methods
Synthetic Routes and Reaction Conditions: Methyl 5-azidopentanoate can be synthesized through several methods. One common approach involves the reaction of 5-bromopentanoic acid methyl ester with sodium azide in the presence of a suitable solvent. The reaction typically occurs under reflux conditions, leading to the substitution of the bromine atom with an azido group .
Industrial Production Methods: In industrial settings, the synthesis of this compound often involves large-scale batch reactions. The process includes the careful control of reaction parameters such as temperature, pressure, and solvent choice to ensure high yield and purity. The use of continuous flow reactors has also been explored to enhance production efficiency and safety .
Chemical Reactions Analysis
Types of Reactions: Methyl 5-azidopentanoate undergoes various chemical reactions, including:
Reduction: The azido group can be reduced to an amine using reducing agents such as hydrogen gas in the presence of a palladium catalyst.
Substitution: The azido group can participate in nucleophilic substitution reactions, forming new carbon-nitrogen bonds.
Cycloaddition: The azido group can undergo cycloaddition reactions, such as the Huisgen 1,3-dipolar cycloaddition, to form triazoles.
Common Reagents and Conditions:
Reduction: Hydrogen gas, palladium catalyst, and suitable solvents.
Substitution: Sodium azide, suitable solvents, and reflux conditions.
Cycloaddition: Alkynes, copper catalysts, and appropriate solvents.
Major Products Formed:
Reduction: Formation of 5-aminopentanoic acid methyl ester.
Substitution: Formation of various substituted pentanoic acid methyl esters.
Cycloaddition: Formation of triazole derivatives.
Scientific Research Applications
Methyl 5-azidopentanoate has diverse applications in scientific research:
Chemistry: It serves as a precursor in the synthesis of other organic compounds, particularly in click chemistry for the formation of triazoles.
Biology: It is used in bioconjugation techniques to label biomolecules with azido groups, facilitating the study of biological processes.
Medicine: The compound is explored for its potential in drug development, particularly in the synthesis of bioactive molecules.
Industry: It is used in the production of specialty chemicals and materials, including polymers and coatings.
Mechanism of Action
The mechanism of action of Methyl 5-azidopentanoate primarily involves its azido group. The azido group is highly reactive and can participate in various chemical reactions, such as cycloaddition and reduction. In biological systems, the azido group can be reduced to an amine, which can then interact with biomolecules, leading to various biological effects .
Comparison with Similar Compounds
- Methyl 5-azidovalerate
- 5-azidovaleric acid methyl ester
- Pentanoic acid, 5-azido-, methyl ester
Comparison: Methyl 5-azidopentanoate is unique due to its specific structure and reactivity. Compared to similar compounds, it offers distinct advantages in terms of reactivity and versatility in chemical synthesis. Its azido group provides a unique handle for various chemical transformations, making it a valuable compound in both research and industrial applications .
Properties
IUPAC Name |
methyl 5-azidopentanoate | |
---|---|---|
Source | PubChem | |
URL | https://pubchem.ncbi.nlm.nih.gov | |
Description | Data deposited in or computed by PubChem | |
InChI |
InChI=1S/C6H11N3O2/c1-11-6(10)4-2-3-5-8-9-7/h2-5H2,1H3 | |
Source | PubChem | |
URL | https://pubchem.ncbi.nlm.nih.gov | |
Description | Data deposited in or computed by PubChem | |
InChI Key |
OQEZMRVNSROGPF-UHFFFAOYSA-N | |
Source | PubChem | |
URL | https://pubchem.ncbi.nlm.nih.gov | |
Description | Data deposited in or computed by PubChem | |
Canonical SMILES |
COC(=O)CCCCN=[N+]=[N-] | |
Source | PubChem | |
URL | https://pubchem.ncbi.nlm.nih.gov | |
Description | Data deposited in or computed by PubChem | |
Molecular Formula |
C6H11N3O2 | |
Source | PubChem | |
URL | https://pubchem.ncbi.nlm.nih.gov | |
Description | Data deposited in or computed by PubChem | |
DSSTOX Substance ID |
DTXSID00561681 | |
Record name | Methyl 5-azidopentanoate | |
Source | EPA DSSTox | |
URL | https://comptox.epa.gov/dashboard/DTXSID00561681 | |
Description | DSSTox provides a high quality public chemistry resource for supporting improved predictive toxicology. | |
Molecular Weight |
157.17 g/mol | |
Source | PubChem | |
URL | https://pubchem.ncbi.nlm.nih.gov | |
Description | Data deposited in or computed by PubChem | |
CAS No. |
127811-93-2 | |
Record name | Methyl 5-azidopentanoate | |
Source | EPA DSSTox | |
URL | https://comptox.epa.gov/dashboard/DTXSID00561681 | |
Description | DSSTox provides a high quality public chemistry resource for supporting improved predictive toxicology. | |
Synthesis routes and methods I
Procedure details
Synthesis routes and methods II
Procedure details
Retrosynthesis Analysis
AI-Powered Synthesis Planning: Our tool employs the Template_relevance Pistachio, Template_relevance Bkms_metabolic, Template_relevance Pistachio_ringbreaker, Template_relevance Reaxys, Template_relevance Reaxys_biocatalysis model, leveraging a vast database of chemical reactions to predict feasible synthetic routes.
One-Step Synthesis Focus: Specifically designed for one-step synthesis, it provides concise and direct routes for your target compounds, streamlining the synthesis process.
Accurate Predictions: Utilizing the extensive PISTACHIO, BKMS_METABOLIC, PISTACHIO_RINGBREAKER, REAXYS, REAXYS_BIOCATALYSIS database, our tool offers high-accuracy predictions, reflecting the latest in chemical research and data.
Strategy Settings
Precursor scoring | Relevance Heuristic |
---|---|
Min. plausibility | 0.01 |
Model | Template_relevance |
Template Set | Pistachio/Bkms_metabolic/Pistachio_ringbreaker/Reaxys/Reaxys_biocatalysis |
Top-N result to add to graph | 6 |
Feasible Synthetic Routes
Disclaimer and Information on In-Vitro Research Products
Please be aware that all articles and product information presented on BenchChem are intended solely for informational purposes. The products available for purchase on BenchChem are specifically designed for in-vitro studies, which are conducted outside of living organisms. In-vitro studies, derived from the Latin term "in glass," involve experiments performed in controlled laboratory settings using cells or tissues. It is important to note that these products are not categorized as medicines or drugs, and they have not received approval from the FDA for the prevention, treatment, or cure of any medical condition, ailment, or disease. We must emphasize that any form of bodily introduction of these products into humans or animals is strictly prohibited by law. It is essential to adhere to these guidelines to ensure compliance with legal and ethical standards in research and experimentation.