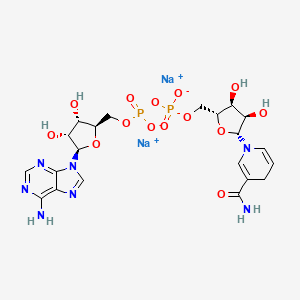
Disodium nicotinamide adenine dinucleotide
- Click on QUICK INQUIRY to receive a quote from our team of experts.
- With the quality product at a COMPETITIVE price, you can focus more on your research.
Overview
Description
Preparation Methods
Synthetic Routes and Reaction Conditions: NADH Disodium Salt Hydrate is typically synthesized through enzymatic reduction of NAD+ (nicotinamide adenine dinucleotide) using specific dehydrogenases such as malic dehydrogenase and lactic dehydrogenase. These enzymes catalyze the reversible transfer of a hydride ion from substrates like malate or lactate to NAD+, forming NADH .
Industrial Production Methods: In industrial settings, NADH Disodium Salt Hydrate is produced through fermentation processes involving microorganisms that naturally produce NADH. The compound is then extracted and purified using various chromatographic techniques to achieve high purity levels .
Chemical Reactions Analysis
Types of Reactions: NADH Disodium Salt Hydrate primarily undergoes oxidation-reduction reactions. It acts as a reducing agent, donating electrons to various substrates in metabolic pathways .
Common Reagents and Conditions:
Oxidation: NADH can be oxidized back to NAD+ using oxidizing agents such as oxygen or other electron acceptors in the presence of dehydrogenases.
Reduction: NADH is formed by the reduction of NAD+ using hydride donors in enzymatic reactions.
Major Products: The major product of NADH oxidation is NAD+, while the reduction of NAD+ results in the formation of NADH .
Scientific Research Applications
NADH Disodium Salt Hydrate has a wide range of applications in scientific research:
Mechanism of Action
NADH Disodium Salt Hydrate functions as a coenzyme for a large number of oxidoreductases. It participates in catabolic processes by donating electrons, which are transferred through the electron transport chain to produce ATP, the primary energy currency of the cell. NADH also plays a role in cell signaling events, such as the DNA damage response, by serving as a substrate for poly (ADP-ribose) polymerases (PARPs) .
Comparison with Similar Compounds
NAD+ (Nicotinamide Adenine Dinucleotide): The oxidized form of NADH, involved in similar metabolic processes but acts as an electron acceptor.
NADPH (Nicotinamide Adenine Dinucleotide Phosphate): Functions similarly to NADH but is primarily involved in anabolic reactions and biosynthetic pathways.
FADH2 (Flavin Adenine Dinucleotide, Reduced): Another electron carrier involved in the electron transport chain but differs in its structure and specific roles in metabolism.
Uniqueness: NADH Disodium Salt Hydrate is unique due to its specific role as an electron donor in catabolic processes and its involvement in various cellular signaling pathways. Its reduced form allows it to participate in redox reactions essential for energy production and metabolic regulation .
Properties
CAS No. |
606-68-8 |
---|---|
Molecular Formula |
C21H27N7Na2O14P2 |
Molecular Weight |
709.4 g/mol |
IUPAC Name |
disodium;[[(2R,3S,4R,5R)-5-(6-aminopurin-9-yl)-3,4-dihydroxyoxolan-2-yl]methoxy-oxidophosphoryl] [(2R,3S,4R,5R)-5-(3-carbamoyl-4H-pyridin-1-yl)-3,4-dihydroxyoxolan-2-yl]methyl phosphate |
InChI |
InChI=1S/C21H29N7O14P2.2Na/c22-17-12-19(25-7-24-17)28(8-26-12)21-16(32)14(30)11(41-21)6-39-44(36,37)42-43(34,35)38-5-10-13(29)15(31)20(40-10)27-3-1-2-9(4-27)18(23)33;;/h1,3-4,7-8,10-11,13-16,20-21,29-32H,2,5-6H2,(H2,23,33)(H,34,35)(H,36,37)(H2,22,24,25);;/q;2*+1/p-2/t10-,11-,13-,14-,15-,16-,20-,21-;;/m1../s1 |
InChI Key |
QRGNQKGQENGQSE-WUEGHLCSSA-L |
Isomeric SMILES |
C1C=CN(C=C1C(=O)N)[C@H]2[C@@H]([C@@H]([C@H](O2)COP(=O)([O-])OP(=O)([O-])OC[C@@H]3[C@H]([C@H]([C@@H](O3)N4C=NC5=C(N=CN=C54)N)O)O)O)O.[Na+].[Na+] |
SMILES |
C1C=CN(C=C1C(=O)N)C2C(C(C(O2)COP(=O)([O-])OP(=O)([O-])OCC3C(C(C(O3)N4C=NC5=C(N=CN=C54)N)O)O)O)O.[Na+].[Na+] |
Canonical SMILES |
C1C=CN(C=C1C(=O)N)C2C(C(C(O2)COP(=O)([O-])OP(=O)([O-])OCC3C(C(C(O3)N4C=NC5=C(N=CN=C54)N)O)O)O)O.[Na+].[Na+] |
606-68-8 | |
Origin of Product |
United States |
Retrosynthesis Analysis
AI-Powered Synthesis Planning: Our tool employs the Template_relevance Pistachio, Template_relevance Bkms_metabolic, Template_relevance Pistachio_ringbreaker, Template_relevance Reaxys, Template_relevance Reaxys_biocatalysis model, leveraging a vast database of chemical reactions to predict feasible synthetic routes.
One-Step Synthesis Focus: Specifically designed for one-step synthesis, it provides concise and direct routes for your target compounds, streamlining the synthesis process.
Accurate Predictions: Utilizing the extensive PISTACHIO, BKMS_METABOLIC, PISTACHIO_RINGBREAKER, REAXYS, REAXYS_BIOCATALYSIS database, our tool offers high-accuracy predictions, reflecting the latest in chemical research and data.
Strategy Settings
Precursor scoring | Relevance Heuristic |
---|---|
Min. plausibility | 0.01 |
Model | Template_relevance |
Template Set | Pistachio/Bkms_metabolic/Pistachio_ringbreaker/Reaxys/Reaxys_biocatalysis |
Top-N result to add to graph | 6 |
Feasible Synthetic Routes
Disclaimer and Information on In-Vitro Research Products
Please be aware that all articles and product information presented on BenchChem are intended solely for informational purposes. The products available for purchase on BenchChem are specifically designed for in-vitro studies, which are conducted outside of living organisms. In-vitro studies, derived from the Latin term "in glass," involve experiments performed in controlled laboratory settings using cells or tissues. It is important to note that these products are not categorized as medicines or drugs, and they have not received approval from the FDA for the prevention, treatment, or cure of any medical condition, ailment, or disease. We must emphasize that any form of bodily introduction of these products into humans or animals is strictly prohibited by law. It is essential to adhere to these guidelines to ensure compliance with legal and ethical standards in research and experimentation.