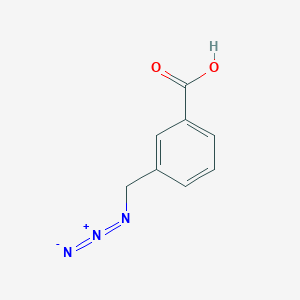
3-(Azidomethyl)benzoic acid
Overview
Description
3-(Azidomethyl)benzoic acid is an organic compound with the molecular formula C8H7N3O2 It is characterized by the presence of an azidomethyl group attached to the benzene ring of benzoic acid
Mechanism of Action
Target of Action
It’s known that the compound exhibits conformational polymorphism , which suggests it may interact with various targets in different conformations.
Mode of Action
Its conformational polymorphism suggests that it can exist in multiple conformations and potentially interact with its targets in different ways . This polymorphism is primarily attributed to the azidomethyl group, which could have implications in the design of solid-state reactions .
Biochemical Pathways
It’s worth noting that benzoic acid, a related compound, is involved in the core β-oxidative pathway in plants
Result of Action
Its conformational polymorphism suggests that it could have diverse effects depending on its conformation .
Action Environment
The environment can significantly influence the action, efficacy, and stability of 3-(Azidomethyl)benzoic acid. Factors such as temperature, pH, and the presence of other molecules could potentially affect its conformation and, consequently, its interactions with targets .
Biochemical Analysis
Biochemical Properties
3-(Azidomethyl)benzoic acid plays a significant role in biochemical reactions, particularly in click chemistry. Click chemistry is a class of biocompatible reactions that are used to join small units together quickly and reliably. The azide group in this compound can undergo copper-catalyzed azide-alkyne cycloaddition (CuAAC) with molecules containing alkyne groups, forming stable triazole linkages . This reaction is highly specific and efficient, making this compound a valuable reagent in the synthesis of complex biomolecules.
In addition to its role in click chemistry, this compound interacts with various enzymes and proteins. For instance, it can be used to label proteins through bioorthogonal reactions, allowing researchers to study protein interactions and functions in a cellular context . The nature of these interactions is typically covalent, resulting in stable conjugates that can be analyzed using various biochemical techniques.
Cellular Effects
This compound has been shown to influence various cellular processes. Its ability to participate in click chemistry reactions allows it to be used as a tool for studying cell signaling pathways, gene expression, and cellular metabolism. For example, by labeling specific proteins or nucleic acids with this compound, researchers can track the localization and dynamics of these molecules within cells .
Furthermore, this compound can affect cell function by modifying the activity of key signaling proteins. This can lead to changes in gene expression patterns and alterations in cellular metabolism. The compound’s impact on cell signaling pathways can be studied using techniques such as fluorescence microscopy and mass spectrometry, providing insights into its role in cellular processes .
Molecular Mechanism
The molecular mechanism of action of this compound involves its ability to form covalent bonds with biomolecules through click chemistry reactions. The azide group in this compound reacts with alkyne-containing molecules in the presence of a copper catalyst, resulting in the formation of triazole linkages . This reaction is highly specific and efficient, allowing for precise labeling of biomolecules.
In addition to its role in click chemistry, this compound can interact with enzymes and proteins, leading to enzyme inhibition or activation. For example, it can inhibit the activity of certain enzymes by covalently modifying their active sites, thereby altering their catalytic properties . These interactions can result in changes in gene expression and cellular function, providing insights into the compound’s molecular mechanism of action.
Temporal Effects in Laboratory Settings
The effects of this compound in laboratory settings can change over time due to factors such as stability and degradation. Studies have shown that the compound is relatively stable under standard laboratory conditions, but it can degrade over time when exposed to light or heat . This degradation can lead to a decrease in its effectiveness in biochemical reactions and cellular assays.
Long-term effects of this compound on cellular function have been observed in both in vitro and in vivo studies. For example, prolonged exposure to the compound can result in changes in cell morphology and alterations in gene expression patterns . These effects can be monitored using techniques such as real-time PCR and Western blotting, providing valuable information on the compound’s temporal effects in laboratory settings.
Dosage Effects in Animal Models
The effects of this compound can vary with different dosages in animal models. Studies have shown that low doses of the compound can have minimal effects on animal physiology, while higher doses can lead to toxic or adverse effects . For example, high doses of this compound have been associated with liver toxicity and changes in metabolic parameters in animal models .
Threshold effects have also been observed, where a certain dosage level is required to elicit a significant biological response. These threshold effects can be studied using dose-response experiments, allowing researchers to determine the optimal dosage for specific applications . Understanding the dosage effects of this compound is crucial for its safe and effective use in biochemical research.
Metabolic Pathways
This compound is involved in various metabolic pathways, including those related to its degradation and excretion. The compound can be metabolized by enzymes such as cytochrome P450, leading to the formation of metabolites that are excreted from the body . These metabolic pathways can be studied using techniques such as mass spectrometry and nuclear magnetic resonance (NMR) spectroscopy, providing insights into the compound’s fate in biological systems.
In addition to its degradation, this compound can also affect metabolic flux and metabolite levels. For example, it can alter the levels of key metabolites involved in energy production and cellular respiration . These effects can be studied using metabolomics approaches, allowing researchers to understand the compound’s impact on cellular metabolism.
Transport and Distribution
The transport and distribution of this compound within cells and tissues are influenced by various factors, including its interactions with transporters and binding proteins. The compound can be transported across cell membranes by specific transporters, allowing it to reach its target sites within cells . Once inside the cell, this compound can interact with binding proteins that facilitate its localization and accumulation in specific cellular compartments .
The distribution of this compound within tissues can also be influenced by factors such as blood flow and tissue permeability. For example, the compound can accumulate in tissues with high blood flow, such as the liver and kidneys . Understanding the transport and distribution of this compound is crucial for its effective use in biochemical research and therapeutic applications.
Subcellular Localization
The subcellular localization of this compound can affect its activity and function. The compound can be directed to specific cellular compartments through targeting signals or post-translational modifications . For example, it can be localized to the cytoplasm, nucleus, or mitochondria, depending on the presence of specific targeting sequences .
The subcellular localization of this compound can be studied using techniques such as fluorescence microscopy and subcellular fractionation. These techniques allow researchers to visualize the compound’s distribution within cells and determine its effects on cellular function . Understanding the subcellular localization of this compound is essential for elucidating its role in cellular processes and biochemical reactions.
Preparation Methods
Synthetic Routes and Reaction Conditions: The synthesis of 3-(azidomethyl)benzoic acid typically involves the introduction of an azido group to the benzoic acid framework. One common method is the reaction of 3-(bromomethyl)benzoic acid with sodium azide in a polar solvent such as dimethylformamide (DMF) at elevated temperatures. The reaction proceeds via nucleophilic substitution, where the bromine atom is replaced by the azido group.
Industrial Production Methods: Industrial production of this compound may involve similar synthetic routes but on a larger scale. The process would be optimized for yield and purity, often involving continuous flow reactors and advanced purification techniques to ensure the consistent quality of the final product.
Chemical Reactions Analysis
Types of Reactions: 3-(Azidomethyl)benzoic acid undergoes various chemical reactions, including:
Oxidation: The azido group can be oxidized to form nitro or nitroso derivatives under specific conditions.
Reduction: Reduction of the azido group can yield amines or amides.
Substitution: The azido group can participate in substitution reactions, such as the Staudinger reaction, where it reacts with phosphines to form iminophosphoranes.
Common Reagents and Conditions:
Oxidation: Reagents like hydrogen peroxide or peracids can be used.
Reduction: Catalysts such as palladium on carbon (Pd/C) or hydrogen gas are commonly employed.
Substitution: Triphenylphosphine (PPh3) is often used in the Staudinger reaction.
Major Products:
Oxidation: Nitrobenzoic acid derivatives.
Reduction: Aminobenzoic acid derivatives.
Substitution: Iminophosphorane derivatives.
Scientific Research Applications
3-(Azidomethyl)benzoic acid has diverse applications in scientific research:
Chemistry: It is used as a precursor in the synthesis of various heterocyclic compounds and as a building block in organic synthesis.
Biology: The compound is utilized in bioconjugation techniques, where it helps in attaching biomolecules to surfaces or other molecules.
Industry: The compound is used in the production of specialty chemicals and materials with specific properties.
Comparison with Similar Compounds
3-(Bromomethyl)benzoic acid: A precursor in the synthesis of 3-(azidomethyl)benzoic acid.
3-(Chloromethyl)benzoic acid: Another halomethyl derivative with similar reactivity.
3-(Hydroxymethyl)benzoic acid: A compound with a hydroxyl group instead of an azido group.
Uniqueness: this compound is unique due to its azido group, which imparts distinct reactivity and versatility in chemical synthesis. The azido group allows for specific reactions, such as click chemistry, which are not possible with other similar compounds.
Properties
IUPAC Name |
3-(azidomethyl)benzoic acid | |
---|---|---|
Source | PubChem | |
URL | https://pubchem.ncbi.nlm.nih.gov | |
Description | Data deposited in or computed by PubChem | |
InChI |
InChI=1S/C8H7N3O2/c9-11-10-5-6-2-1-3-7(4-6)8(12)13/h1-4H,5H2,(H,12,13) | |
Source | PubChem | |
URL | https://pubchem.ncbi.nlm.nih.gov | |
Description | Data deposited in or computed by PubChem | |
InChI Key |
YHRRDHYXBMYXQF-UHFFFAOYSA-N | |
Source | PubChem | |
URL | https://pubchem.ncbi.nlm.nih.gov | |
Description | Data deposited in or computed by PubChem | |
Canonical SMILES |
C1=CC(=CC(=C1)C(=O)O)CN=[N+]=[N-] | |
Source | PubChem | |
URL | https://pubchem.ncbi.nlm.nih.gov | |
Description | Data deposited in or computed by PubChem | |
Molecular Formula |
C8H7N3O2 | |
Source | PubChem | |
URL | https://pubchem.ncbi.nlm.nih.gov | |
Description | Data deposited in or computed by PubChem | |
Molecular Weight |
177.16 g/mol | |
Source | PubChem | |
URL | https://pubchem.ncbi.nlm.nih.gov | |
Description | Data deposited in or computed by PubChem | |
Retrosynthesis Analysis
AI-Powered Synthesis Planning: Our tool employs the Template_relevance Pistachio, Template_relevance Bkms_metabolic, Template_relevance Pistachio_ringbreaker, Template_relevance Reaxys, Template_relevance Reaxys_biocatalysis model, leveraging a vast database of chemical reactions to predict feasible synthetic routes.
One-Step Synthesis Focus: Specifically designed for one-step synthesis, it provides concise and direct routes for your target compounds, streamlining the synthesis process.
Accurate Predictions: Utilizing the extensive PISTACHIO, BKMS_METABOLIC, PISTACHIO_RINGBREAKER, REAXYS, REAXYS_BIOCATALYSIS database, our tool offers high-accuracy predictions, reflecting the latest in chemical research and data.
Strategy Settings
Precursor scoring | Relevance Heuristic |
---|---|
Min. plausibility | 0.01 |
Model | Template_relevance |
Template Set | Pistachio/Bkms_metabolic/Pistachio_ringbreaker/Reaxys/Reaxys_biocatalysis |
Top-N result to add to graph | 6 |
Feasible Synthetic Routes
Q1: What makes 3-(azidomethyl)benzoic acid prone to polymorphism?
A1: [] The research highlights the azidomethyl group (-CH2N3) within the this compound molecule as the primary driver of its conformational polymorphism. This is evidenced by the three distinct crystal structures observed, all exhibiting similar carboxylic acid dimer and π–π stacking arrangements, but differing in the conformation of the azidomethyl group. This suggests that the azidomethyl group introduces conformational flexibility, leading to different packing arrangements in the crystal lattice and thus polymorphism.
Q2: What are the potential implications of this compound's polymorphism?
A2: The existence of multiple polymorphs of this compound is significant because different polymorphs can exhibit varying physical and chemical properties, such as solubility, stability, and reactivity. [] This has important implications for potential applications, especially in the context of solid-state reactions. For instance, different polymorphs may react at different rates or even lead to different reaction products. Therefore, understanding and controlling the polymorphic behavior of this compound is crucial for its successful utilization in various applications.
Disclaimer and Information on In-Vitro Research Products
Please be aware that all articles and product information presented on BenchChem are intended solely for informational purposes. The products available for purchase on BenchChem are specifically designed for in-vitro studies, which are conducted outside of living organisms. In-vitro studies, derived from the Latin term "in glass," involve experiments performed in controlled laboratory settings using cells or tissues. It is important to note that these products are not categorized as medicines or drugs, and they have not received approval from the FDA for the prevention, treatment, or cure of any medical condition, ailment, or disease. We must emphasize that any form of bodily introduction of these products into humans or animals is strictly prohibited by law. It is essential to adhere to these guidelines to ensure compliance with legal and ethical standards in research and experimentation.