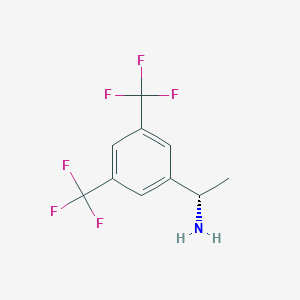
(S)-1-(3,5-Bis(trifluoromethyl)phenyl)ethanamine
Overview
Description
(S)-1-(3,5-Bis(trifluoromethyl)phenyl)ethanamine is a chiral amine compound characterized by the presence of two trifluoromethyl groups attached to a phenyl ring. This compound is of significant interest in various fields of chemistry due to its unique structural features and reactivity.
Mechanism of Action
Target of Action
Compounds with similar structures, such as n,n′-bis[3,5-bis(trifluoromethyl)phenyl]thiourea, have been used as organocatalysts in organic chemistry . They activate substrates and stabilize partially developing negative charges (e.g., oxyanions) in the transition states .
Mode of Action
Similar compounds have been shown to activate substrates and stabilize partially developing negative charges (eg, oxyanions) in the transition states . This is achieved through explicit double hydrogen bonding .
Biochemical Pathways
Similar compounds have been used extensively in promoting organic transformations .
Pharmacokinetics
Similar compounds have been synthesized via the asymmetric reduction of 3,5-bis(trifluoromethyl) acetophenone, catalyzed by leifsonia xyli cctcc m 2010241 cells using isopropanol as the co-substrate for cofactor recycling .
Action Environment
Similar compounds have been synthesized under mild conditions, with a broad substrate scope and excellent functional group compatibility .
Preparation Methods
Synthetic Routes and Reaction Conditions
The synthesis of (S)-1-(3,5-Bis(trifluoromethyl)phenyl)ethanamine typically involves the enantioselective reduction of the corresponding ketone precursor. One common method is the use of engineered ketoreductases (KREDS) for the highly enantiospecific reduction of prochiral ketones . This process can be carried out using whole microbial cells or isolated enzymes, and the reaction conditions often include the use of specific cofactors and controlled temperature and pH conditions.
Industrial Production Methods
Industrial production of this compound may involve large-scale biocatalytic processes utilizing immobilized enzymes to enhance the efficiency and yield of the desired enantiomer. The use of continuous flow reactors and advanced purification techniques ensures the high purity and scalability of the production process.
Chemical Reactions Analysis
Types of Reactions
(S)-1-(3,5-Bis(trifluoromethyl)phenyl)ethanamine undergoes various types of chemical reactions, including:
Oxidation: The compound can be oxidized to form the corresponding imine or nitrile derivatives.
Reduction: Reduction reactions can convert the compound into different amine derivatives.
Substitution: Nucleophilic substitution reactions can introduce various functional groups onto the phenyl ring or the amine moiety.
Common Reagents and Conditions
Common reagents used in these reactions include oxidizing agents like potassium permanganate, reducing agents such as lithium aluminum hydride, and nucleophiles like alkyl halides. Reaction conditions typically involve controlled temperatures, inert atmospheres, and specific solvents to ensure the desired selectivity and yield.
Major Products Formed
The major products formed from these reactions include imines, nitriles, secondary amines, and various substituted phenyl derivatives, depending on the specific reaction conditions and reagents used.
Scientific Research Applications
(S)-1-(3,5-Bis(trifluoromethyl)phenyl)ethanamine has a wide range of scientific research applications:
Chemistry: It is used as a building block in the synthesis of complex organic molecules and as a ligand in coordination chemistry.
Biology: The compound is studied for its potential biological activity and interactions with biomolecules.
Medicine: Research explores its potential as a pharmaceutical intermediate and its role in drug development.
Industry: It is utilized in the production of specialty chemicals and materials with unique properties.
Comparison with Similar Compounds
Similar Compounds
N,N′-Bis(3,5-bis(trifluoromethyl)phenyl)thiourea: Known for its role as a catalyst in organic reactions.
3,5-Bis(trifluoromethyl)phenyl-substituted iridium complexes: Used in materials science for their unique emission properties.
Uniqueness
(S)-1-(3,5-Bis(trifluoromethyl)phenyl)ethanamine is unique due to its chiral nature and the presence of two trifluoromethyl groups, which impart distinct electronic and steric properties. These features make it a valuable compound in asymmetric synthesis and catalysis.
Properties
IUPAC Name |
(1S)-1-[3,5-bis(trifluoromethyl)phenyl]ethanamine | |
---|---|---|
Source | PubChem | |
URL | https://pubchem.ncbi.nlm.nih.gov | |
Description | Data deposited in or computed by PubChem | |
InChI |
InChI=1S/C10H9F6N/c1-5(17)6-2-7(9(11,12)13)4-8(3-6)10(14,15)16/h2-5H,17H2,1H3/t5-/m0/s1 | |
Source | PubChem | |
URL | https://pubchem.ncbi.nlm.nih.gov | |
Description | Data deposited in or computed by PubChem | |
InChI Key |
PFVWEAYXWZFSSK-YFKPBYRVSA-N | |
Source | PubChem | |
URL | https://pubchem.ncbi.nlm.nih.gov | |
Description | Data deposited in or computed by PubChem | |
Canonical SMILES |
CC(C1=CC(=CC(=C1)C(F)(F)F)C(F)(F)F)N | |
Source | PubChem | |
URL | https://pubchem.ncbi.nlm.nih.gov | |
Description | Data deposited in or computed by PubChem | |
Isomeric SMILES |
C[C@@H](C1=CC(=CC(=C1)C(F)(F)F)C(F)(F)F)N | |
Source | PubChem | |
URL | https://pubchem.ncbi.nlm.nih.gov | |
Description | Data deposited in or computed by PubChem | |
Molecular Formula |
C10H9F6N | |
Source | PubChem | |
URL | https://pubchem.ncbi.nlm.nih.gov | |
Description | Data deposited in or computed by PubChem | |
DSSTOX Substance ID |
DTXSID10363693 | |
Record name | (1S)-1-[3,5-Bis(trifluoromethyl)phenyl]ethan-1-amine | |
Source | EPA DSSTox | |
URL | https://comptox.epa.gov/dashboard/DTXSID10363693 | |
Description | DSSTox provides a high quality public chemistry resource for supporting improved predictive toxicology. | |
Molecular Weight |
257.18 g/mol | |
Source | PubChem | |
URL | https://pubchem.ncbi.nlm.nih.gov | |
Description | Data deposited in or computed by PubChem | |
CAS No. |
127733-40-8 | |
Record name | (1S)-1-[3,5-Bis(trifluoromethyl)phenyl]ethan-1-amine | |
Source | EPA DSSTox | |
URL | https://comptox.epa.gov/dashboard/DTXSID10363693 | |
Description | DSSTox provides a high quality public chemistry resource for supporting improved predictive toxicology. | |
Synthesis routes and methods I
Procedure details
Synthesis routes and methods II
Procedure details
Disclaimer and Information on In-Vitro Research Products
Please be aware that all articles and product information presented on BenchChem are intended solely for informational purposes. The products available for purchase on BenchChem are specifically designed for in-vitro studies, which are conducted outside of living organisms. In-vitro studies, derived from the Latin term "in glass," involve experiments performed in controlled laboratory settings using cells or tissues. It is important to note that these products are not categorized as medicines or drugs, and they have not received approval from the FDA for the prevention, treatment, or cure of any medical condition, ailment, or disease. We must emphasize that any form of bodily introduction of these products into humans or animals is strictly prohibited by law. It is essential to adhere to these guidelines to ensure compliance with legal and ethical standards in research and experimentation.