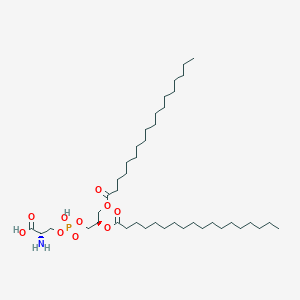
Phosphatidylserine
Overview
Description
Phosphatidylserine is a phospholipid that plays a crucial role in the structure and function of cell membranes. It is predominantly found in the inner leaflet of the plasma membrane and is essential for various cellular processes, including cell signaling and apoptosis. This compound is composed of a glycerol backbone, two fatty acid chains, a phosphate group, and the amino acid serine .
Mechanism of Action
Target of Action
Distearoylphosphatidylserine (DSPS), also known as Phosphatidylethanolamine, primarily targets immune cells . It is preferentially expressed by immune cells and is known to regulate inflammation, autoimmune diseases, and cancer . DSPS acts as a monolayer and serves as the “eat-me” signal for professional phagocytes such as macrophages and neutrophils of the immune system .
Mode of Action
DSPS interacts with its targets, the immune cells, by acting as a monolayer . This interaction leads to changes in the immune response, specifically in the regulation of inflammation, autoimmune diseases, and cancer .
Biochemical Pathways
The biochemical pathways affected by DSPS are primarily related to immune response regulation . The compound plays a vital role in several intracellular signaling pathways . Many pathways contribute to the cellular metabolism of DSPS, with parallels but also differences between yeast and mammalian cells .
Pharmacokinetics
It is known that dsps-containing nanoparticles improve their uptake by immune cells
Result of Action
The result of DSPS action is the inhibition of c-Rel expression in myeloid and lymphoid cells . This leads to a decrease in inflammation, autoimmune diseases, and cancer . The compound’s action could be exploited to target immune cells to limit the development of inflammatory diseases or cancer caused by c-Rel upregulation .
Action Environment
The action environment of DSPS is primarily within the immune system, where it interacts with immune cells . Environmental factors that could influence the compound’s action, efficacy, and stability include the presence of other immune cells, the state of the immune system, and the overall health of the individual.
Biochemical Analysis
Biochemical Properties
Distearoylphosphatidylserine interacts with various enzymes, proteins, and other biomolecules. It plays a vital role in several intracellular signaling pathways . The function of Distearoylthis compound, as with all lipids, is determined by both its concentration and sidedness in individual organellar membranes .
Cellular Effects
Distearoylthis compound influences cell function, including impacts on cell signaling pathways, gene expression, and cellular metabolism . It is generally not externally exposed in healthy cells . It clearly plays a vital role in healthy cells .
Molecular Mechanism
The mechanism of action of Distearoylthis compound involves its interactions with biomolecules and changes in gene expression . The topology of Distearoylthis compound results from the actions of transmembrane enzymes capable of moving it between lipid bilayers .
Temporal Effects in Laboratory Settings
It is known that the bulk subcellular distribution of Distearoylthis compound results from the coordinated actions of metabolic enzymes in conjunction with vesicular and nonvesicular transport pathways .
Metabolic Pathways
Distearoylthis compound is involved in several metabolic pathways. The bulk subcellular distribution of Distearoylthis compound results from the coordinated actions of metabolic enzymes in conjunction with vesicular and nonvesicular transport pathways .
Transport and Distribution
Distearoylthis compound is transported and distributed within cells and tissues. Mitochondria associated membranes (MAMs) of the endoplasmic reticulum (ER) have high rates of Distearoylthis compound synthesis and serve as a conduit for the transfer of lipids between the ER and adjacent mitochondria .
Subcellular Localization
It is known that Distearoylthis compound is more concentrated in the plasma membrane with lower levels in the ER and mitochondria .
Preparation Methods
Synthetic Routes and Reaction Conditions: Phosphatidylserine can be synthesized through enzymatic catalytic reactions. One common method involves the use of phospholipase D to catalyze the reaction between natural lecithin and L-serine. This reaction typically occurs in the presence of an organic solvent and an inorganic salt, resulting in the formation of this compound .
Industrial Production Methods: In industrial settings, this compound is often produced using a similar enzymatic process. The reaction involves mixing lecithin with L-serine and phospholipase D in an aqueous solution. The mixture is then emulsified, and the reaction is allowed to proceed under controlled conditions. After the reaction is complete, the product is purified through extraction and chromatography techniques .
Chemical Reactions Analysis
Types of Reactions: Phosphatidylserine undergoes various chemical reactions, including hydrolysis, oxidation, and substitution.
Common Reagents and Conditions:
Hydrolysis: This reaction involves the cleavage of the phosphodiester bond in this compound, resulting in the formation of phosphatidic acid and serine.
Oxidation: this compound can undergo oxidation reactions, particularly in the presence of reactive oxygen species.
Major Products: The major products formed from these reactions include phosphatidic acid, serine, and various oxidized phospholipid species .
Scientific Research Applications
Phosphatidylserine has a wide range of applications in scientific research, particularly in the fields of chemistry, biology, medicine, and industry.
Chemistry: In chemistry, this compound is used as a model compound to study the properties and behavior of phospholipids in biological membranes .
Biology: In biological research, this compound is studied for its role in cell signaling, apoptosis, and membrane dynamics. It is also used as a marker for apoptotic cells, as its externalization on the cell surface signals the cell for phagocytosis .
Medicine: this compound is investigated for its potential therapeutic applications, particularly in the treatment of neurodegenerative diseases such as Alzheimer’s disease. It is believed to enhance cognitive function and memory by supporting neuronal cell membrane integrity and signaling pathways .
Industry: In the food and pharmaceutical industries, this compound is used as a dietary supplement to promote cognitive health and reduce stress .
Comparison with Similar Compounds
Phosphatidylethanolamine: Another phospholipid found in cell membranes, involved in membrane fusion and cell signaling.
Phosphatidylcholine: A major component of biological membranes, important for membrane structure and function.
Sphingomyelin: A phospholipid found in the myelin sheath of nerve cells, involved in signal transduction and cell recognition.
Phosphatidylserine’s unique properties and functions make it a valuable compound for research and therapeutic applications.
Properties
IUPAC Name |
(2S)-2-amino-3-[[(2R)-2,3-di(octadecanoyloxy)propoxy]-hydroxyphosphoryl]oxypropanoic acid | |
---|---|---|
Source | PubChem | |
URL | https://pubchem.ncbi.nlm.nih.gov | |
Description | Data deposited in or computed by PubChem | |
InChI |
InChI=1S/C42H82NO10P/c1-3-5-7-9-11-13-15-17-19-21-23-25-27-29-31-33-40(44)50-35-38(36-51-54(48,49)52-37-39(43)42(46)47)53-41(45)34-32-30-28-26-24-22-20-18-16-14-12-10-8-6-4-2/h38-39H,3-37,43H2,1-2H3,(H,46,47)(H,48,49)/t38-,39+/m1/s1 | |
Source | PubChem | |
URL | https://pubchem.ncbi.nlm.nih.gov | |
Description | Data deposited in or computed by PubChem | |
InChI Key |
TZCPCKNHXULUIY-RGULYWFUSA-N | |
Source | PubChem | |
URL | https://pubchem.ncbi.nlm.nih.gov | |
Description | Data deposited in or computed by PubChem | |
Canonical SMILES |
CCCCCCCCCCCCCCCCCC(=O)OCC(COP(=O)(O)OCC(C(=O)O)N)OC(=O)CCCCCCCCCCCCCCCCC | |
Source | PubChem | |
URL | https://pubchem.ncbi.nlm.nih.gov | |
Description | Data deposited in or computed by PubChem | |
Isomeric SMILES |
CCCCCCCCCCCCCCCCCC(=O)OC[C@H](COP(=O)(O)OC[C@@H](C(=O)O)N)OC(=O)CCCCCCCCCCCCCCCCC | |
Source | PubChem | |
URL | https://pubchem.ncbi.nlm.nih.gov | |
Description | Data deposited in or computed by PubChem | |
Molecular Formula |
C42H82NO10P | |
Source | PubChem | |
URL | https://pubchem.ncbi.nlm.nih.gov | |
Description | Data deposited in or computed by PubChem | |
DSSTOX Substance ID |
DTXSID10866199 | |
Record name | 1,2-Distearoyl phosphatidyl serine | |
Source | EPA DSSTox | |
URL | https://comptox.epa.gov/dashboard/DTXSID10866199 | |
Description | DSSTox provides a high quality public chemistry resource for supporting improved predictive toxicology. | |
Molecular Weight |
792.1 g/mol | |
Source | PubChem | |
URL | https://pubchem.ncbi.nlm.nih.gov | |
Description | Data deposited in or computed by PubChem | |
Physical Description |
Solid | |
Record name | PS(18:0/18:0) | |
Source | Human Metabolome Database (HMDB) | |
URL | http://www.hmdb.ca/metabolites/HMDB0012378 | |
Description | The Human Metabolome Database (HMDB) is a freely available electronic database containing detailed information about small molecule metabolites found in the human body. | |
Explanation | HMDB is offered to the public as a freely available resource. Use and re-distribution of the data, in whole or in part, for commercial purposes requires explicit permission of the authors and explicit acknowledgment of the source material (HMDB) and the original publication (see the HMDB citing page). We ask that users who download significant portions of the database cite the HMDB paper in any resulting publications. | |
CAS No. |
1446756-47-3, 51446-62-9 | |
Record name | Phosphatidyl serine | |
Source | ChemIDplus | |
URL | https://pubchem.ncbi.nlm.nih.gov/substance/?source=chemidplus&sourceid=1446756473 | |
Description | ChemIDplus is a free, web search system that provides access to the structure and nomenclature authority files used for the identification of chemical substances cited in National Library of Medicine (NLM) databases, including the TOXNET system. | |
Record name | 1,2-Distearoyl phosphatidyl serine | |
Source | EPA DSSTox | |
URL | https://comptox.epa.gov/dashboard/DTXSID10866199 | |
Description | DSSTox provides a high quality public chemistry resource for supporting improved predictive toxicology. | |
Record name | PHOSPHATIDYL SERINE | |
Source | FDA Global Substance Registration System (GSRS) | |
URL | https://gsrs.ncats.nih.gov/ginas/app/beta/substances/394XK0IH40 | |
Description | The FDA Global Substance Registration System (GSRS) enables the efficient and accurate exchange of information on what substances are in regulated products. Instead of relying on names, which vary across regulatory domains, countries, and regions, the GSRS knowledge base makes it possible for substances to be defined by standardized, scientific descriptions. | |
Explanation | Unless otherwise noted, the contents of the FDA website (www.fda.gov), both text and graphics, are not copyrighted. They are in the public domain and may be republished, reprinted and otherwise used freely by anyone without the need to obtain permission from FDA. Credit to the U.S. Food and Drug Administration as the source is appreciated but not required. | |
Retrosynthesis Analysis
AI-Powered Synthesis Planning: Our tool employs the Template_relevance Pistachio, Template_relevance Bkms_metabolic, Template_relevance Pistachio_ringbreaker, Template_relevance Reaxys, Template_relevance Reaxys_biocatalysis model, leveraging a vast database of chemical reactions to predict feasible synthetic routes.
One-Step Synthesis Focus: Specifically designed for one-step synthesis, it provides concise and direct routes for your target compounds, streamlining the synthesis process.
Accurate Predictions: Utilizing the extensive PISTACHIO, BKMS_METABOLIC, PISTACHIO_RINGBREAKER, REAXYS, REAXYS_BIOCATALYSIS database, our tool offers high-accuracy predictions, reflecting the latest in chemical research and data.
Strategy Settings
Precursor scoring | Relevance Heuristic |
---|---|
Min. plausibility | 0.01 |
Model | Template_relevance |
Template Set | Pistachio/Bkms_metabolic/Pistachio_ringbreaker/Reaxys/Reaxys_biocatalysis |
Top-N result to add to graph | 6 |
Feasible Synthetic Routes
Disclaimer and Information on In-Vitro Research Products
Please be aware that all articles and product information presented on BenchChem are intended solely for informational purposes. The products available for purchase on BenchChem are specifically designed for in-vitro studies, which are conducted outside of living organisms. In-vitro studies, derived from the Latin term "in glass," involve experiments performed in controlled laboratory settings using cells or tissues. It is important to note that these products are not categorized as medicines or drugs, and they have not received approval from the FDA for the prevention, treatment, or cure of any medical condition, ailment, or disease. We must emphasize that any form of bodily introduction of these products into humans or animals is strictly prohibited by law. It is essential to adhere to these guidelines to ensure compliance with legal and ethical standards in research and experimentation.