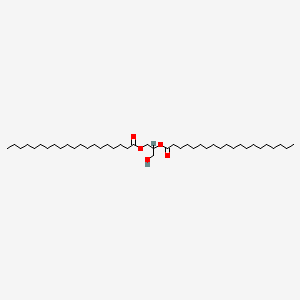
(3-Hydroxy-2-icosanoyloxypropyl) icosanoate
Overview
Description
(3-Hydroxy-2-icosanoyloxypropyl) icosanoate (HIPI) is a unique biocompatible compound that has been used in a variety of scientific research applications. HIPI is a powerful antioxidant and has been used as a therapeutic agent for many diseases. HIPI has been used in the synthesis of various polymers, as well as in the development of nanomaterials. HIPI has also been used in the production of biodegradable polymers and in the development of drug delivery systems.
Scientific Research Applications
Lipid Metabolism and Inflammatory Response
- Inflammation and Lipid Peroxides : The study by Terao and Matsushita (1981) examined arachidonic acid, closely related to icosanoic acid, and its oxidation products. They found that these lipid peroxides can play a role in various biological processes, including inflammatory responses (Terao & Matsushita, 1981).
Bioactive Compounds and Inflammation
- Resolvins from Omega-3 Fatty Acids : Serhan et al. (2002) reported on resolvins, a family of bioactive compounds derived from omega-3 fatty acids like DHA and EPA, which can counter proinflammatory signals. This research suggests a potential application in controlling inflammation (Serhan et al., 2002).
Neutrophil Responses and Chemotaxis
- Neutrophil Cell Responses : Norgauer et al. (1996) explored the responses of neutrophils to chemotactic arachidonic acid metabolites, indicating a unique pattern of neutrophil responses and signaling pathways. This could be relevant to understanding immune responses and designing therapeutics targeting such pathways (Norgauer et al., 1996).
Free Radical Scavenging and Anti-Inflammatory Activity
- Free Radical Scavenging : The study by Bonne et al. (1990) investigated CBS-113 A, a compound with free radical scavenging and anti-inflammatory properties. It highlights the potential therapeutic applications of compounds with antioxidant properties in inflammation-related diseases (Bonne et al., 1990).
Arachidonic Acid Metabolism
- Platelet Aggregation and Icosanoid Formation : Krug and Berndt (1987) studied the effect of triethyl lead chloride on platelet aggregation and the formation of icosanoids. This research is significant for understanding how various substances can affect platelet function and the metabolism of arachidonic acid, a related compound to icosanoic acid (Krug & Berndt, 1987).
properties
IUPAC Name |
(3-hydroxy-2-icosanoyloxypropyl) icosanoate | |
---|---|---|
Source | PubChem | |
URL | https://pubchem.ncbi.nlm.nih.gov | |
Description | Data deposited in or computed by PubChem | |
InChI |
InChI=1S/C43H84O5/c1-3-5-7-9-11-13-15-17-19-21-23-25-27-29-31-33-35-37-42(45)47-40-41(39-44)48-43(46)38-36-34-32-30-28-26-24-22-20-18-16-14-12-10-8-6-4-2/h41,44H,3-40H2,1-2H3 | |
Source | PubChem | |
URL | https://pubchem.ncbi.nlm.nih.gov | |
Description | Data deposited in or computed by PubChem | |
InChI Key |
AGUTXIBMYVFOMK-UHFFFAOYSA-N | |
Source | PubChem | |
URL | https://pubchem.ncbi.nlm.nih.gov | |
Description | Data deposited in or computed by PubChem | |
Canonical SMILES |
CCCCCCCCCCCCCCCCCCCC(=O)OCC(CO)OC(=O)CCCCCCCCCCCCCCCCCCC | |
Source | PubChem | |
URL | https://pubchem.ncbi.nlm.nih.gov | |
Description | Data deposited in or computed by PubChem | |
Molecular Formula |
C43H84O5 | |
Source | PubChem | |
URL | https://pubchem.ncbi.nlm.nih.gov | |
Description | Data deposited in or computed by PubChem | |
Molecular Weight |
681.1 g/mol | |
Source | PubChem | |
URL | https://pubchem.ncbi.nlm.nih.gov | |
Description | Data deposited in or computed by PubChem | |
Product Name |
(3-Hydroxy-2-icosanoyloxypropyl) icosanoate |
Retrosynthesis Analysis
AI-Powered Synthesis Planning: Our tool employs the Template_relevance Pistachio, Template_relevance Bkms_metabolic, Template_relevance Pistachio_ringbreaker, Template_relevance Reaxys, Template_relevance Reaxys_biocatalysis model, leveraging a vast database of chemical reactions to predict feasible synthetic routes.
One-Step Synthesis Focus: Specifically designed for one-step synthesis, it provides concise and direct routes for your target compounds, streamlining the synthesis process.
Accurate Predictions: Utilizing the extensive PISTACHIO, BKMS_METABOLIC, PISTACHIO_RINGBREAKER, REAXYS, REAXYS_BIOCATALYSIS database, our tool offers high-accuracy predictions, reflecting the latest in chemical research and data.
Strategy Settings
Precursor scoring | Relevance Heuristic |
---|---|
Min. plausibility | 0.01 |
Model | Template_relevance |
Template Set | Pistachio/Bkms_metabolic/Pistachio_ringbreaker/Reaxys/Reaxys_biocatalysis |
Top-N result to add to graph | 6 |
Feasible Synthetic Routes
Disclaimer and Information on In-Vitro Research Products
Please be aware that all articles and product information presented on BenchChem are intended solely for informational purposes. The products available for purchase on BenchChem are specifically designed for in-vitro studies, which are conducted outside of living organisms. In-vitro studies, derived from the Latin term "in glass," involve experiments performed in controlled laboratory settings using cells or tissues. It is important to note that these products are not categorized as medicines or drugs, and they have not received approval from the FDA for the prevention, treatment, or cure of any medical condition, ailment, or disease. We must emphasize that any form of bodily introduction of these products into humans or animals is strictly prohibited by law. It is essential to adhere to these guidelines to ensure compliance with legal and ethical standards in research and experimentation.