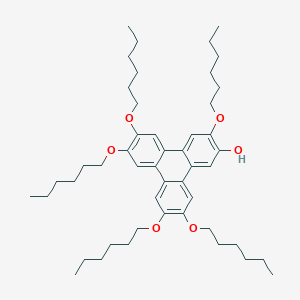
2-Hydroxy-3,6,7,10,11-pentakis(hexyloxy)triphenylene
Overview
Description
2-Hydroxy-3,6,7,10,11-pentakis(hexyloxy)triphenylene: is a complex organic compound with the molecular formula C48H72O6 It is a derivative of triphenylene, a polycyclic aromatic hydrocarbon, and is characterized by the presence of five hexyloxy groups and a hydroxyl group attached to the triphenylene core
Mechanism of Action
Target of Action
The primary target of the compound 2-Hydroxy-3,6,7,10,11-pentakis(hexyloxy)triphenylene, also known as 3,6,7,10,11-Pentahexoxytriphenylen-2-ol, is the formation of liquid crystal structures . This compound is known to form columnar liquid crystal phases , which are stable over a wide temperature interval .
Mode of Action
The mode of action of this compound involves the formation of these liquid crystal structures through oxidative coupling . The compound interacts with its targets by forming 1:1 CPI (complementary polytopic interaction) compounds with other triphenylene derivatives . These additives can induce liquid crystal behavior in otherwise non-mesogenic esters .
Biochemical Pathways
The biochemical pathway of this compound involves the oxidative coupling of 2-acetoxy-1-hexyloxybenzene to 3,3′,4,4′-tetrakis(hexyloxy)biphenyl . This process leads to the formation of the compound, which then forms an enantiotopic Col h columnar liquid crystal phase .
Pharmacokinetics
It is known that the compound forms stable liquid crystal phases over a wide temperature range , suggesting that it may have good thermal stability.
Result of Action
The result of the action of this compound is the formation of stable liquid crystal structures . These structures can be further enhanced by the formation of 1:1 CPI compounds with other triphenylene derivatives .
Action Environment
The action of this compound is influenced by environmental factors such as temperature . The compound forms stable liquid crystal phases over a wide temperature range , suggesting that it may be robust to changes in environmental temperature.
Preparation Methods
Synthetic Routes and Reaction Conditions: The synthesis of 2-Hydroxy-3,6,7,10,11-pentakis(hexyloxy)triphenylene typically involves the following steps:
Starting Materials: The synthesis begins with 1,2-dihexyloxybenzene and 1,1’-biphenyl, 3,3’,4,4’-tetrakis(hexyloxy)-.
Reaction Conditions: The reaction is carried out under controlled conditions, often involving the use of solvents such as acetonitrile and catalysts like molybdenum pentachloride.
Oxidative Trimerization: The key step involves the oxidative trimerization of catechol derivatives, which can be induced by metal salts in high oxidation states or through electrochemical methods.
Industrial Production Methods: Industrial production of this compound may involve large-scale electroorganic synthesis. This method is preferred due to its efficiency and the ability to produce high-purity compounds. The process involves anodic treatment of catechol ketals followed by acidic hydrolysis .
Chemical Reactions Analysis
Types of Reactions: 2-Hydroxy-3,6,7,10,11-pentakis(hexyloxy)triphenylene undergoes various chemical reactions, including:
Oxidation: The compound can be oxidized to form higher oxidation states.
Substitution: The hexyloxy groups can be substituted with other functional groups under appropriate conditions.
Common Reagents and Conditions:
Oxidation: Reagents such as molybdenum pentachloride or electrochemical methods are commonly used.
Substitution: Reagents like halogens or alkylating agents can be used for substitution reactions.
Major Products:
Oxidation Products: Higher oxidation states of the compound.
Substitution Products: Compounds with different functional groups replacing the hexyloxy groups.
Scientific Research Applications
2-Hydroxy-3,6,7,10,11-pentakis(hexyloxy)triphenylene has a wide range of applications in scientific research:
Biology: Potential use in the development of biosensors due to its unique structural properties.
Medicine: Investigated for its potential use in drug delivery systems.
Industry: Used in the production of materials with high thermal stability and unique optical properties.
Comparison with Similar Compounds
2,3,6,7,10,11-Hexahydroxytriphenylene: Similar in structure but with hydroxyl groups instead of hexyloxy groups.
2,3,6,7,10,11-Hexamethoxytriphenylene: Contains methoxy groups instead of hexyloxy groups.
2,3,6,7,10,11-Hexahydroxybenzophenone: Another derivative with different functional groups.
Uniqueness: 2-Hydroxy-3,6,7,10,11-pentakis(hexyloxy)triphenylene is unique due to its combination of hexyloxy groups and a hydroxyl group, which imparts distinct physical and chemical properties. Its ability to form stable columnar mesophases and its high thermal stability make it particularly valuable in the field of organic electronics and materials science .
Properties
IUPAC Name |
3,6,7,10,11-pentahexoxytriphenylen-2-ol | |
---|---|---|
Source | PubChem | |
URL | https://pubchem.ncbi.nlm.nih.gov | |
Description | Data deposited in or computed by PubChem | |
InChI |
InChI=1S/C48H72O6/c1-6-11-16-21-26-50-44-32-38-37(31-43(44)49)39-33-45(51-27-22-17-12-7-2)47(53-29-24-19-14-9-4)35-41(39)42-36-48(54-30-25-20-15-10-5)46(34-40(38)42)52-28-23-18-13-8-3/h31-36,49H,6-30H2,1-5H3 | |
Source | PubChem | |
URL | https://pubchem.ncbi.nlm.nih.gov | |
Description | Data deposited in or computed by PubChem | |
InChI Key |
IUYYUSKHZOXORT-UHFFFAOYSA-N | |
Source | PubChem | |
URL | https://pubchem.ncbi.nlm.nih.gov | |
Description | Data deposited in or computed by PubChem | |
Canonical SMILES |
CCCCCCOC1=C(C=C2C(=C1)C3=CC(=C(C=C3C4=CC(=C(C=C24)OCCCCCC)OCCCCCC)OCCCCCC)OCCCCCC)O | |
Source | PubChem | |
URL | https://pubchem.ncbi.nlm.nih.gov | |
Description | Data deposited in or computed by PubChem | |
Molecular Formula |
C48H72O6 | |
Source | PubChem | |
URL | https://pubchem.ncbi.nlm.nih.gov | |
Description | Data deposited in or computed by PubChem | |
Molecular Weight |
745.1 g/mol | |
Source | PubChem | |
URL | https://pubchem.ncbi.nlm.nih.gov | |
Description | Data deposited in or computed by PubChem | |
Synthesis routes and methods
Procedure details
Retrosynthesis Analysis
AI-Powered Synthesis Planning: Our tool employs the Template_relevance Pistachio, Template_relevance Bkms_metabolic, Template_relevance Pistachio_ringbreaker, Template_relevance Reaxys, Template_relevance Reaxys_biocatalysis model, leveraging a vast database of chemical reactions to predict feasible synthetic routes.
One-Step Synthesis Focus: Specifically designed for one-step synthesis, it provides concise and direct routes for your target compounds, streamlining the synthesis process.
Accurate Predictions: Utilizing the extensive PISTACHIO, BKMS_METABOLIC, PISTACHIO_RINGBREAKER, REAXYS, REAXYS_BIOCATALYSIS database, our tool offers high-accuracy predictions, reflecting the latest in chemical research and data.
Strategy Settings
Precursor scoring | Relevance Heuristic |
---|---|
Min. plausibility | 0.01 |
Model | Template_relevance |
Template Set | Pistachio/Bkms_metabolic/Pistachio_ringbreaker/Reaxys/Reaxys_biocatalysis |
Top-N result to add to graph | 6 |
Feasible Synthetic Routes
Disclaimer and Information on In-Vitro Research Products
Please be aware that all articles and product information presented on BenchChem are intended solely for informational purposes. The products available for purchase on BenchChem are specifically designed for in-vitro studies, which are conducted outside of living organisms. In-vitro studies, derived from the Latin term "in glass," involve experiments performed in controlled laboratory settings using cells or tissues. It is important to note that these products are not categorized as medicines or drugs, and they have not received approval from the FDA for the prevention, treatment, or cure of any medical condition, ailment, or disease. We must emphasize that any form of bodily introduction of these products into humans or animals is strictly prohibited by law. It is essential to adhere to these guidelines to ensure compliance with legal and ethical standards in research and experimentation.