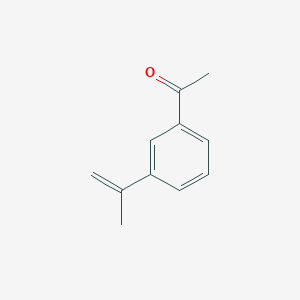
1-(3-Prop-1-en-2-ylphenyl)ethanone
- Click on QUICK INQUIRY to receive a quote from our team of experts.
- With the quality product at a COMPETITIVE price, you can focus more on your research.
Overview
Description
1-(3-Prop-1-en-2-ylphenyl)ethanone is a useful research compound. Its molecular formula is C11H12O and its molecular weight is 160.21 g/mol. The purity is usually 95%.
BenchChem offers high-quality this compound suitable for many research applications. Different packaging options are available to accommodate customers' requirements. Please inquire for more information about this compound including the price, delivery time, and more detailed information at info@benchchem.com.
Q & A
Basic Research Questions
Q. What are the optimal synthetic routes for 1-(3-Prop-1-en-2-ylphenyl)ethanone, and how do reaction conditions influence yield?
- Methodological Answer : The Friedel-Crafts acylation is a common method for synthesizing aromatic ketones like this compound. Use acetyl chloride and a Lewis acid catalyst (e.g., AlCl₃) under anhydrous conditions. Temperature control (0–5°C) minimizes side reactions, while extended reaction times (12–24 hrs) improve yield . Solvent choice (e.g., dichloromethane or nitrobenzene) affects regioselectivity due to steric and electronic effects of the allyl group. Post-reaction, quenching with ice-HCl and purification via column chromatography (silica gel, hexane/ethyl acetate) are critical for isolating the product .
Q. How can structural characterization of this compound be performed to confirm its purity and configuration?
- Methodological Answer : Combine spectroscopic and crystallographic techniques:
- NMR : ¹H and ¹³C NMR identify the allyl group (δ 5.1–5.3 ppm for vinyl protons) and ketone (δ 2.6 ppm for methyl protons). NOESY can confirm spatial proximity of substituents .
- XRD : Single-crystal X-ray diffraction (using SHELX software ) resolves bond angles and distances, critical for verifying the allyl group's orientation and potential π-π stacking interactions.
- MS : High-resolution mass spectrometry (HRMS) validates molecular weight and fragmentation patterns .
Advanced Research Questions
Q. What computational methods are suitable for predicting the reactivity of this compound in electrophilic substitution reactions?
- Methodological Answer : Density functional theory (DFT) with hybrid functionals (e.g., B3LYP ) calculates frontier molecular orbitals (HOMO/LUMO) to predict sites for electrophilic attack. Basis sets like 6-31G(d,p) model electron density around the aromatic ring and allyl group. Solvent effects can be incorporated using the polarizable continuum model (PCM). Compare results with experimental kinetic data (e.g., rate constants from UV-Vis monitoring) to validate computational models .
Q. How do steric and electronic effects of the allyl group influence regioselectivity in derivatization reactions?
- Methodological Answer : The allyl group’s electron-donating resonance effect activates the para position, while steric hindrance directs electrophiles to the ortho/meta positions. Use Hammett substituent constants (σ⁺) to quantify electronic effects. Competitive experiments with substituted analogs (e.g., methyl vs. allyl) under identical conditions (e.g., nitration with HNO₃/H₂SO₄) reveal regioselectivity trends. HPLC or GC-MS monitors product distribution .
Q. What strategies mitigate data discrepancies in biological activity studies of derivatives?
- Methodological Answer : Standardize assay protocols to reduce variability:
- Enzyme assays : Use recombinant enzymes (e.g., CYP450 isoforms) and control for pH, temperature, and cofactors.
- Molecular docking : Employ PyRx or AutoDock Vina with crystal structures from the PDB (e.g., 1MNC for kinases). Validate docking poses via molecular dynamics simulations (GROMACS) .
- ADMET profiling : Use SwissADME to predict bioavailability and rule out false positives from cytotoxicity (e.g., MTT assays on HEK-293 cells) .
Q. How can reaction pathways involving the ketone and allyl groups be selectively controlled?
- Methodological Answer :
- Ketone protection : Convert the ketone to a thioketal using ethanedithiol and BF₃·Et₂O, enabling selective allyl oxidation (e.g., ozonolysis to aldehyde) .
- Cross-metathesis : Employ Grubbs catalyst to functionalize the allyl group without affecting the ketone. Monitor reaction progress via FT-IR for C=C bond disappearance .
Q. Data-Driven Research Challenges
Q. What analytical techniques resolve contradictions in reported crystallographic data for similar ethanone derivatives?
- Methodological Answer : Reanalyze disputed structures using:
- Rietveld refinement : For powder XRD data, refine lattice parameters and occupancy factors to detect impurities.
- SC-XRD : Compare thermal ellipsoids and residual electron density maps to identify disorder or twinning .
- DFT-optimized geometries : Overlay computational and experimental structures to assess fit (RMSD < 0.5 Å acceptable) .
Q. How can solvent effects on NMR chemical shifts be quantified for accurate structural assignments?
- Methodological Answer : Record ¹H NMR in deuterated solvents (CDCl₃, DMSO-d₆) and apply solvent correction factors. Use COSY and HSQC to resolve overlapping signals. For polar solvents, apply the PCM-DFT method to simulate solvent-induced shifts (e.g., Gaussian 16) and compare with experimental data .
Q. Biological and Pharmacological Applications
Q. What in vitro models are appropriate for evaluating the antimicrobial potential of this compound derivatives?
- Methodological Answer : Use standardized MIC (minimum inhibitory concentration) assays against Gram-positive (e.g., S. aureus ATCC 25923) and Gram-negative (e.g., E. coli ATCC 25922) strains. Pair with time-kill kinetics to assess bactericidal vs. bacteriostatic effects. Validate membrane disruption via SEM imaging of treated cells .
Q. How can the compound’s pharmacokinetic profile be optimized for CNS penetration?
Properties
Molecular Formula |
C11H12O |
---|---|
Molecular Weight |
160.21 g/mol |
IUPAC Name |
1-(3-prop-1-en-2-ylphenyl)ethanone |
InChI |
InChI=1S/C11H12O/c1-8(2)10-5-4-6-11(7-10)9(3)12/h4-7H,1H2,2-3H3 |
InChI Key |
CDWRSUNYLNEVTA-UHFFFAOYSA-N |
SMILES |
CC(=C)C1=CC(=CC=C1)C(=O)C |
Canonical SMILES |
CC(=C)C1=CC(=CC=C1)C(=O)C |
Origin of Product |
United States |
Disclaimer and Information on In-Vitro Research Products
Please be aware that all articles and product information presented on BenchChem are intended solely for informational purposes. The products available for purchase on BenchChem are specifically designed for in-vitro studies, which are conducted outside of living organisms. In-vitro studies, derived from the Latin term "in glass," involve experiments performed in controlled laboratory settings using cells or tissues. It is important to note that these products are not categorized as medicines or drugs, and they have not received approval from the FDA for the prevention, treatment, or cure of any medical condition, ailment, or disease. We must emphasize that any form of bodily introduction of these products into humans or animals is strictly prohibited by law. It is essential to adhere to these guidelines to ensure compliance with legal and ethical standards in research and experimentation.