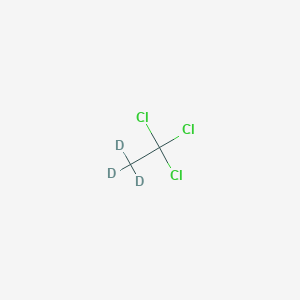
1,1,1-Trichloroethane-2,2,2-d3
Overview
Description
1,1,1-Trichloroethane-2,2,2-d3 is a deuterated form of 1,1,1-trichloroethane, where the hydrogen atoms are replaced with deuterium. This compound is a chloroalkane with the chemical formula CCl3CD3. It is a colorless liquid with a sweet, chloroform-like odor and is used primarily in scientific research due to its unique isotopic properties .
Mechanism of Action
Target of Action
The primary targets of 1,1,1-Trichloroethane-2,2,2-d3 are the central nervous system and the respiratory system . It acts as a central nervous system depressant , and inhalation of its vapors may cause dizziness, drowsiness, headache, nausea, shortness of breath, and unconsciousness .
Mode of Action
The exact mode of action of This compound It is known to cause skin irritation and is harmful if inhaled . It is also known to cause central nervous system depression .
Biochemical Pathways
The biochemical pathways affected by This compound It is known that it can be formed when another chemical breaks down in the environment under conditions where there is no air .
Pharmacokinetics
The ADME (Absorption, Distribution, Metabolism, and Excretion) properties of This compound It is known that it has a high henry’s law constant and will volatilize rapidly from water and soil .
Result of Action
The molecular and cellular effects of This compound include skin irritation and harmful effects if inhaled . It can also cause central nervous system depression, leading to symptoms such as dizziness, drowsiness, headache, nausea, shortness of breath, and unconsciousness .
Action Environment
Environmental factors can influence the action, efficacy, and stability of This compound . For instance, it can evaporate from soil and water when they are exposed to the air . It is also known to be formed when another chemical breaks down in the environment under conditions where there is no air .
Biochemical Analysis
Biochemical Properties
1,1,1-Trichloroethane-2,2,2-d3 plays a significant role in biochemical reactions, particularly in studies involving metabolic pathways and enzyme interactions. It interacts with various enzymes, including those involved in reductive dechlorination. For instance, it can be reductively dechlorinated under anaerobic conditions by enzymes from Desulfobacterium autotrophicum and Clostridium species . These interactions are crucial for understanding the degradation pathways and environmental impact of chlorinated solvents.
Cellular Effects
This compound affects various cellular processes, including cell signaling pathways, gene expression, and cellular metabolism. It has been observed to cause changes in liver enzymes and histopathology in animal studies . Additionally, it can influence central nervous system functions, leading to symptoms such as sleepiness and motor impairment . These effects highlight the compound’s potential impact on cellular health and function.
Molecular Mechanism
The molecular mechanism of this compound involves its interaction with biomolecules through reductive dechlorination and oxidation processes. It can be transformed into 2,2,2-trichloroethanol by methanotrophs like Methylosinus trichosporium, which express soluble methane monooxygenase . This transformation involves binding interactions with enzymes that facilitate the dechlorination and oxidation of the compound, leading to the formation of various metabolites.
Temporal Effects in Laboratory Settings
In laboratory settings, the effects of this compound can change over time due to its stability and degradation. The compound is relatively stable under standard storage conditions but can degrade over time, leading to changes in its biochemical activity . Long-term studies have shown that its effects on cellular function can persist, with potential cumulative impacts on cellular health and metabolism.
Dosage Effects in Animal Models
The effects of this compound vary with different dosages in animal models. At lower doses, it may cause mild changes in liver enzymes and cellular metabolism, while higher doses can lead to more severe effects such as central nervous system depression and liver damage . These dosage-dependent effects are crucial for understanding the compound’s toxicity and safe usage levels.
Metabolic Pathways
This compound is involved in several metabolic pathways, including reductive dechlorination and aerobic oxidation. It can be transformed into 2,2,2-trichloroethanol, trichloroacetic acid, and dichloroacetic acid by various microorganisms . These metabolic pathways are essential for understanding the compound’s environmental fate and potential detoxification mechanisms.
Transport and Distribution
Within cells and tissues, this compound is transported and distributed through interactions with transporters and binding proteins. Its distribution can affect its localization and accumulation in specific tissues, influencing its overall biochemical activity . Understanding these transport mechanisms is vital for predicting the compound’s behavior in biological systems.
Subcellular Localization
This compound can localize to specific subcellular compartments, affecting its activity and function. It may be directed to particular organelles through targeting signals or post-translational modifications, influencing its interactions with cellular components . This subcellular localization is crucial for understanding the compound’s precise biochemical effects.
Preparation Methods
1,1,1-Trichloroethane-2,2,2-d3 can be synthesized through several methods. One common synthetic route involves the chlorination of ethane in the presence of deuterium gas. The reaction typically occurs under controlled conditions to ensure the selective substitution of hydrogen atoms with deuterium. Industrial production methods often involve the use of catalysts to enhance the reaction efficiency and yield .
Chemical Reactions Analysis
1,1,1-Trichloroethane-2,2,2-d3 undergoes various chemical reactions, including:
Substitution Reactions: It can undergo nucleophilic substitution reactions where the chlorine atoms are replaced by other nucleophiles.
Reduction Reactions: The compound can be reduced to form less chlorinated derivatives.
Oxidation Reactions: It can be oxidized to form trichloroacetic acid or other oxidized products.
Common reagents used in these reactions include sodium hydroxide for substitution, hydrogen gas for reduction, and potassium permanganate for oxidation. The major products formed depend on the specific reaction conditions and reagents used .
Scientific Research Applications
1,1,1-Trichloroethane-2,2,2-d3 is widely used in scientific research due to its isotopic labeling, which makes it valuable in various fields:
Chemistry: It is used as a solvent and in the study of reaction mechanisms.
Biology: The compound is used in metabolic studies to trace the pathways of chlorinated compounds in biological systems.
Medicine: It is used in pharmacokinetic studies to understand the distribution and metabolism of chlorinated solvents in the body.
Industry: The compound is used in the development of new materials and in environmental analysis to study the fate of chlorinated compounds
Comparison with Similar Compounds
1,1,1-Trichloroethane-2,2,2-d3 can be compared with other similar compounds such as:
1,1,1-Trichloroethane: The non-deuterated form, which is widely used as a solvent.
1,1,2-Trichloroethane: An isomer with different chemical properties and uses.
1,1-Dichloroethane: A less chlorinated derivative with different reactivity.
The uniqueness of this compound lies in its isotopic labeling, which provides distinct advantages in research applications, particularly in tracing and studying reaction mechanisms .
Properties
IUPAC Name |
1,1,1-trichloro-2,2,2-trideuterioethane | |
---|---|---|
Source | PubChem | |
URL | https://pubchem.ncbi.nlm.nih.gov | |
Description | Data deposited in or computed by PubChem | |
InChI |
InChI=1S/C2H3Cl3/c1-2(3,4)5/h1H3/i1D3 | |
Source | PubChem | |
URL | https://pubchem.ncbi.nlm.nih.gov | |
Description | Data deposited in or computed by PubChem | |
InChI Key |
UOCLXMDMGBRAIB-FIBGUPNXSA-N | |
Source | PubChem | |
URL | https://pubchem.ncbi.nlm.nih.gov | |
Description | Data deposited in or computed by PubChem | |
Canonical SMILES |
CC(Cl)(Cl)Cl | |
Source | PubChem | |
URL | https://pubchem.ncbi.nlm.nih.gov | |
Description | Data deposited in or computed by PubChem | |
Isomeric SMILES |
[2H]C([2H])([2H])C(Cl)(Cl)Cl | |
Source | PubChem | |
URL | https://pubchem.ncbi.nlm.nih.gov | |
Description | Data deposited in or computed by PubChem | |
Molecular Formula |
C2H3Cl3 | |
Source | PubChem | |
URL | https://pubchem.ncbi.nlm.nih.gov | |
Description | Data deposited in or computed by PubChem | |
Molecular Weight |
136.42 g/mol | |
Source | PubChem | |
URL | https://pubchem.ncbi.nlm.nih.gov | |
Description | Data deposited in or computed by PubChem | |
Synthesis routes and methods I
Procedure details
Synthesis routes and methods II
Procedure details
Synthesis routes and methods III
Procedure details
Synthesis routes and methods IV
Procedure details
Retrosynthesis Analysis
AI-Powered Synthesis Planning: Our tool employs the Template_relevance Pistachio, Template_relevance Bkms_metabolic, Template_relevance Pistachio_ringbreaker, Template_relevance Reaxys, Template_relevance Reaxys_biocatalysis model, leveraging a vast database of chemical reactions to predict feasible synthetic routes.
One-Step Synthesis Focus: Specifically designed for one-step synthesis, it provides concise and direct routes for your target compounds, streamlining the synthesis process.
Accurate Predictions: Utilizing the extensive PISTACHIO, BKMS_METABOLIC, PISTACHIO_RINGBREAKER, REAXYS, REAXYS_BIOCATALYSIS database, our tool offers high-accuracy predictions, reflecting the latest in chemical research and data.
Strategy Settings
Precursor scoring | Relevance Heuristic |
---|---|
Min. plausibility | 0.01 |
Model | Template_relevance |
Template Set | Pistachio/Bkms_metabolic/Pistachio_ringbreaker/Reaxys/Reaxys_biocatalysis |
Top-N result to add to graph | 6 |
Feasible Synthetic Routes
Disclaimer and Information on In-Vitro Research Products
Please be aware that all articles and product information presented on BenchChem are intended solely for informational purposes. The products available for purchase on BenchChem are specifically designed for in-vitro studies, which are conducted outside of living organisms. In-vitro studies, derived from the Latin term "in glass," involve experiments performed in controlled laboratory settings using cells or tissues. It is important to note that these products are not categorized as medicines or drugs, and they have not received approval from the FDA for the prevention, treatment, or cure of any medical condition, ailment, or disease. We must emphasize that any form of bodily introduction of these products into humans or animals is strictly prohibited by law. It is essential to adhere to these guidelines to ensure compliance with legal and ethical standards in research and experimentation.