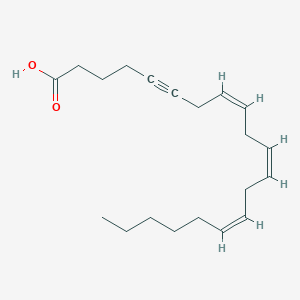
5,6-dehydroarachidonic acid
Overview
Description
5,6-Dehydro Arachidonic Acid is an analog of arachidonic acid, where the 5,6 double bond has been replaced with an acetylene group . This modification results in a compound with unique biochemical properties, making it a valuable tool in scientific research. It is known to inhibit the enzyme 5-lipoxygenase, which plays a crucial role in the metabolism of arachidonic acid .
Mechanism of Action
- Role : 5-LOX is involved in the biosynthesis of leukotrienes, which are potent lipid mediators implicated in inflammation and immune responses .
- Resulting Changes : Reduced leukotriene production may modulate inflammatory processes and cellular responses .
- Affected Pathways : The arachidonic acid pathway is impacted. Specifically, 5,6-dehydroarachidonic acid interferes with the conversion of arachidonic acid to 5-hydroperoxyeicosatetraenoic acid (5-HPETE), a key step in leukotriene synthesis .
Target of Action
Mode of Action
Biochemical Pathways
Biochemical Analysis
Biochemical Properties
5,6-dehydroarachidonic Acid is known to inhibit 5-lipoxygenase (5-LO), an enzyme that plays a crucial role in the biosynthesis of leukotrienes . The inhibition occurs with an IC50 value of 10 μM in guinea pig leukocytes . This interaction with 5-LO suggests that this compound may play a role in modulating inflammatory responses.
Molecular Mechanism
At the molecular level, this compound exerts its effects through its interaction with 5-LO. It binds to this enzyme, inhibiting its activity and thereby reducing the production of leukotrienes . This can lead to changes in cell signaling and potentially impact gene expression related to inflammation and immune response.
Preparation Methods
Synthetic Routes and Reaction Conditions: The synthesis of 5,6-Dehydro Arachidonic Acid typically involves the modification of arachidonic acidThis can be achieved through various chemical reactions, including the use of acetylene derivatives and specific catalysts under controlled conditions .
Industrial Production Methods: Industrial production of 5,6-Dehydro Arachidonic Acid follows similar synthetic routes but on a larger scale. The process involves the use of high-purity reagents and advanced equipment to ensure the consistency and quality of the final product. The compound is usually stored in ethanol solution and shipped under cold conditions to maintain its stability .
Chemical Reactions Analysis
Types of Reactions: 5,6-Dehydro Arachidonic Acid undergoes several types of chemical reactions, including:
Oxidation: The compound can be oxidized to form various oxygenated derivatives.
Reduction: Reduction reactions can convert the acetylene group back to a double bond.
Substitution: The acetylene group can participate in substitution reactions with various nucleophiles.
Common Reagents and Conditions:
Oxidation: Common oxidizing agents include potassium permanganate and chromium trioxide.
Reduction: Hydrogen gas in the presence of a palladium catalyst is often used for reduction.
Substitution: Nucleophiles such as amines and thiols can react with the acetylene group under mild conditions.
Major Products: The major products formed from these reactions depend on the specific conditions and reagents used. For example, oxidation can yield hydroxy derivatives, while reduction can produce alkenes .
Scientific Research Applications
5,6-Dehydro Arachidonic Acid has a wide range of applications in scientific research:
Chemistry: It is used as a model compound to study the reactivity of acetylene groups in fatty acids.
Biology: The compound is employed to investigate the role of 5-lipoxygenase in various biological processes.
Medicine: Research on 5,6-Dehydro Arachidonic Acid contributes to the understanding of inflammatory diseases and the development of anti-inflammatory drugs.
Comparison with Similar Compounds
Arachidonic Acid: The parent compound with a double bond at the 5,6 position.
Eicosapentaenoic Acid: A polyunsaturated fatty acid with multiple double bonds.
Docosahexaenoic Acid: Another polyunsaturated fatty acid with a different structure.
Uniqueness: 5,6-Dehydro Arachidonic Acid is unique due to the presence of an acetylene group at the 5,6 position. This structural modification imparts distinct biochemical properties, such as the ability to inhibit 5-lipoxygenase more effectively than its parent compound, arachidonic acid .
Properties
IUPAC Name |
(8Z,11Z,14Z)-icosa-8,11,14-trien-5-ynoic acid | |
---|---|---|
Source | PubChem | |
URL | https://pubchem.ncbi.nlm.nih.gov | |
Description | Data deposited in or computed by PubChem | |
InChI |
InChI=1S/C20H30O2/c1-2-3-4-5-6-7-8-9-10-11-12-13-14-15-16-17-18-19-20(21)22/h6-7,9-10,12-13H,2-5,8,11,14,17-19H2,1H3,(H,21,22)/b7-6-,10-9-,13-12- | |
Source | PubChem | |
URL | https://pubchem.ncbi.nlm.nih.gov | |
Description | Data deposited in or computed by PubChem | |
InChI Key |
GIOQWSLKUVKKAO-QNEBEIHSSA-N | |
Source | PubChem | |
URL | https://pubchem.ncbi.nlm.nih.gov | |
Description | Data deposited in or computed by PubChem | |
Canonical SMILES |
CCCCCC=CCC=CCC=CCC#CCCCC(=O)O | |
Source | PubChem | |
URL | https://pubchem.ncbi.nlm.nih.gov | |
Description | Data deposited in or computed by PubChem | |
Isomeric SMILES |
CCCCC/C=C\C/C=C\C/C=C\CC#CCCCC(=O)O | |
Source | PubChem | |
URL | https://pubchem.ncbi.nlm.nih.gov | |
Description | Data deposited in or computed by PubChem | |
Molecular Formula |
C20H30O2 | |
Source | PubChem | |
URL | https://pubchem.ncbi.nlm.nih.gov | |
Description | Data deposited in or computed by PubChem | |
Molecular Weight |
302.5 g/mol | |
Source | PubChem | |
URL | https://pubchem.ncbi.nlm.nih.gov | |
Description | Data deposited in or computed by PubChem | |
CAS No. |
58688-54-3 | |
Record name | Eicosa-8,11,14-trien-5-ynoic acid | |
Source | ChemIDplus | |
URL | https://pubchem.ncbi.nlm.nih.gov/substance/?source=chemidplus&sourceid=0058688543 | |
Description | ChemIDplus is a free, web search system that provides access to the structure and nomenclature authority files used for the identification of chemical substances cited in National Library of Medicine (NLM) databases, including the TOXNET system. | |
Retrosynthesis Analysis
AI-Powered Synthesis Planning: Our tool employs the Template_relevance Pistachio, Template_relevance Bkms_metabolic, Template_relevance Pistachio_ringbreaker, Template_relevance Reaxys, Template_relevance Reaxys_biocatalysis model, leveraging a vast database of chemical reactions to predict feasible synthetic routes.
One-Step Synthesis Focus: Specifically designed for one-step synthesis, it provides concise and direct routes for your target compounds, streamlining the synthesis process.
Accurate Predictions: Utilizing the extensive PISTACHIO, BKMS_METABOLIC, PISTACHIO_RINGBREAKER, REAXYS, REAXYS_BIOCATALYSIS database, our tool offers high-accuracy predictions, reflecting the latest in chemical research and data.
Strategy Settings
Precursor scoring | Relevance Heuristic |
---|---|
Min. plausibility | 0.01 |
Model | Template_relevance |
Template Set | Pistachio/Bkms_metabolic/Pistachio_ringbreaker/Reaxys/Reaxys_biocatalysis |
Top-N result to add to graph | 6 |
Feasible Synthetic Routes
Q1: What is 5,6-dehydroarachidonic acid and how does it work?
A1: this compound (also known as 5,6-DHA or Eicosa-8,11,14-trien-5-ynoic acid) is a potent and irreversible inhibitor of 5-lipoxygenase (5-LO), a key enzyme in the arachidonic acid cascade. [, ] This cascade is responsible for producing various signaling molecules involved in inflammation and cell growth. 5,6-DHA acts by binding to the active site of 5-LO, preventing the enzyme from converting arachidonic acid into its downstream products, primarily leukotrienes. [, ]
Q2: What are the downstream effects of inhibiting 5-LO with 5,6-DHA?
A2: By inhibiting 5-LO, 5,6-DHA effectively reduces the production of leukotrienes, specifically leukotriene C4 (LTC4) and leukotriene B4 (LTB4). [] These leukotrienes are potent inflammatory mediators involved in various physiological processes like smooth muscle contraction, vascular permeability, and immune cell recruitment. [] Consequently, 5,6-DHA exhibits anti-inflammatory effects by suppressing leukotriene-mediated signaling pathways.
Q3: How does 5,6-DHA impact cell growth?
A3: Studies have shown that 5,6-DHA can inhibit the growth of various cell types, including vascular smooth muscle cells and endothelial cells. [, ] This effect is attributed to its ability to block the production of 5-LO metabolites, which are suggested to play a role in cell proliferation signaling. []
Q4: Has 5,6-DHA been used to study specific cell types?
A4: Yes, 5,6-DHA has been utilized in research to investigate the role of 5-LO in various cell types, including:
- Fibroblasts: Research suggests that platelet-stimulated proliferation of fibroblasts is mediated by increased 5-LO activity, and this effect can be blocked by 5,6-DHA. []
- Mast cells: Studies have demonstrated that 5,6-DHA can inhibit the release of leukotrienes from mast cells activated by various stimuli, highlighting its role in modulating mast cell degranulation and inflammatory responses. []
- Neurons: 5,6-DHA has been shown to inhibit TRPM7 channel activity, a channel involved in neuronal cell death. This suggests a potential neuroprotective effect of 5,6-DHA. []
Q5: Are there any structural analogs of 5,6-DHA that have different effects?
A5: Yes, while 5,6-DHA specifically targets 5-LO, other structurally similar compounds like 11,12-dehydroarachidonic acid have been shown to irreversibly inhibit the cyclooxygenase pathway, which is responsible for prostaglandin synthesis. [] This highlights the importance of subtle structural variations in achieving selectivity for different enzymes within the arachidonic acid cascade.
Q6: What are the limitations of using 5,6-DHA in research?
A6: While a valuable tool in research, 5,6-DHA has limitations:
Disclaimer and Information on In-Vitro Research Products
Please be aware that all articles and product information presented on BenchChem are intended solely for informational purposes. The products available for purchase on BenchChem are specifically designed for in-vitro studies, which are conducted outside of living organisms. In-vitro studies, derived from the Latin term "in glass," involve experiments performed in controlled laboratory settings using cells or tissues. It is important to note that these products are not categorized as medicines or drugs, and they have not received approval from the FDA for the prevention, treatment, or cure of any medical condition, ailment, or disease. We must emphasize that any form of bodily introduction of these products into humans or animals is strictly prohibited by law. It is essential to adhere to these guidelines to ensure compliance with legal and ethical standards in research and experimentation.