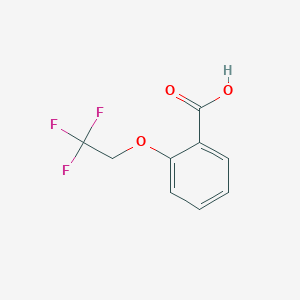
2-(2,2,2-Trifluoroethoxy)benzoic acid
Overview
Description
2-(2,2,2-Trifluoroethoxy)benzoic acid is an organic compound characterized by the presence of a trifluoroethoxy group attached to a benzoic acid moiety. This compound is known for its unique chemical properties, which make it valuable in various scientific and industrial applications. Its molecular formula is C9H7F3O3, and it has a molecular weight of 220.15 g/mol .
Preparation Methods
Synthetic Routes and Reaction Conditions
The synthesis of 2-(2,2,2-Trifluoroethoxy)benzoic acid typically involves the reaction of 2,2,2-trifluoroethanol with a suitable benzoic acid derivative. One common method includes the reaction of 2,2,2-trifluoroethanol with 5-bromo-2-chlorobenzoic acid in the presence of a base to form the desired product . The reaction conditions often involve the use of solvents such as dichloromethane or trichloroethylene and may require the presence of a Lewis acid catalyst .
Industrial Production Methods
Industrial production of this compound follows similar synthetic routes but on a larger scale. The process involves the use of cost-effective starting materials and optimized reaction conditions to ensure high yields and purity of the final product . The scalability of the synthesis process is crucial for its application in various industries.
Chemical Reactions Analysis
Types of Reactions
2-(2,2,2-Trifluoroethoxy)benzoic acid undergoes several types of chemical reactions, including:
Oxidation: The compound can be oxidized to form trifluoroacetic acid.
Substitution: It can participate in nucleophilic substitution reactions, where the trifluoroethoxy group can be replaced by other nucleophiles.
Common Reagents and Conditions
Common reagents used in the reactions of this compound include oxidizing agents like potassium permanganate and nucleophiles such as amines and alcohols. The reaction conditions vary depending on the desired product but often involve the use of solvents like dichloromethane and catalysts such as Lewis acids .
Major Products Formed
The major products formed from the reactions of this compound include trifluoroacetic acid (from oxidation) and various substituted benzoic acid derivatives (from substitution reactions) .
Scientific Research Applications
2-(2,2,2-Trifluoroethoxy)benzoic acid has a wide range of scientific research applications, including:
Mechanism of Action
The mechanism of action of 2-(2,2,2-Trifluoroethoxy)benzoic acid involves its interaction with specific molecular targets, such as enzymes and receptors. The trifluoroethoxy group enhances the compound’s ability to form hydrogen bonds and interact with hydrophobic pockets in proteins, thereby modulating their activity . This interaction can lead to the inhibition or activation of various biochemical pathways, depending on the specific target .
Comparison with Similar Compounds
Similar Compounds
Similar compounds to 2-(2,2,2-Trifluoroethoxy)benzoic acid include:
- 2-(2,2,2-Trifluoroethoxy)phenylboronic acid
- 3-(2,2,2-Trifluoroethoxy)phenylboronic acid
- [4-(2,2,2-Trifluoroethoxy)phenyl]acetic acid
Uniqueness
What sets this compound apart from these similar compounds is its specific structural configuration, which imparts unique chemical properties such as higher reactivity in nucleophilic substitution reactions and greater stability under oxidative conditions . These properties make it particularly valuable in the synthesis of complex organic molecules and pharmaceutical intermediates .
Properties
IUPAC Name |
2-(2,2,2-trifluoroethoxy)benzoic acid | |
---|---|---|
Source | PubChem | |
URL | https://pubchem.ncbi.nlm.nih.gov | |
Description | Data deposited in or computed by PubChem | |
InChI |
InChI=1S/C9H7F3O3/c10-9(11,12)5-15-7-4-2-1-3-6(7)8(13)14/h1-4H,5H2,(H,13,14) | |
Source | PubChem | |
URL | https://pubchem.ncbi.nlm.nih.gov | |
Description | Data deposited in or computed by PubChem | |
InChI Key |
HNVXZKNHBRXZSH-UHFFFAOYSA-N | |
Source | PubChem | |
URL | https://pubchem.ncbi.nlm.nih.gov | |
Description | Data deposited in or computed by PubChem | |
Canonical SMILES |
C1=CC=C(C(=C1)C(=O)O)OCC(F)(F)F | |
Source | PubChem | |
URL | https://pubchem.ncbi.nlm.nih.gov | |
Description | Data deposited in or computed by PubChem | |
Molecular Formula |
C9H7F3O3 | |
Source | PubChem | |
URL | https://pubchem.ncbi.nlm.nih.gov | |
Description | Data deposited in or computed by PubChem | |
Molecular Weight |
220.14 g/mol | |
Source | PubChem | |
URL | https://pubchem.ncbi.nlm.nih.gov | |
Description | Data deposited in or computed by PubChem | |
Synthesis routes and methods I
Procedure details
Synthesis routes and methods II
Procedure details
Synthesis routes and methods III
Procedure details
Retrosynthesis Analysis
AI-Powered Synthesis Planning: Our tool employs the Template_relevance Pistachio, Template_relevance Bkms_metabolic, Template_relevance Pistachio_ringbreaker, Template_relevance Reaxys, Template_relevance Reaxys_biocatalysis model, leveraging a vast database of chemical reactions to predict feasible synthetic routes.
One-Step Synthesis Focus: Specifically designed for one-step synthesis, it provides concise and direct routes for your target compounds, streamlining the synthesis process.
Accurate Predictions: Utilizing the extensive PISTACHIO, BKMS_METABOLIC, PISTACHIO_RINGBREAKER, REAXYS, REAXYS_BIOCATALYSIS database, our tool offers high-accuracy predictions, reflecting the latest in chemical research and data.
Strategy Settings
Precursor scoring | Relevance Heuristic |
---|---|
Min. plausibility | 0.01 |
Model | Template_relevance |
Template Set | Pistachio/Bkms_metabolic/Pistachio_ringbreaker/Reaxys/Reaxys_biocatalysis |
Top-N result to add to graph | 6 |
Feasible Synthetic Routes
Disclaimer and Information on In-Vitro Research Products
Please be aware that all articles and product information presented on BenchChem are intended solely for informational purposes. The products available for purchase on BenchChem are specifically designed for in-vitro studies, which are conducted outside of living organisms. In-vitro studies, derived from the Latin term "in glass," involve experiments performed in controlled laboratory settings using cells or tissues. It is important to note that these products are not categorized as medicines or drugs, and they have not received approval from the FDA for the prevention, treatment, or cure of any medical condition, ailment, or disease. We must emphasize that any form of bodily introduction of these products into humans or animals is strictly prohibited by law. It is essential to adhere to these guidelines to ensure compliance with legal and ethical standards in research and experimentation.