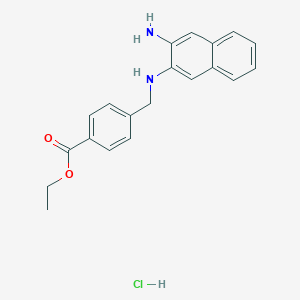
DAN-1 EE hydrochloride
Overview
Description
Preparation Methods
Synthetic Routes and Reaction Conditions
The synthesis of DAN-1 EE (hydrochloride) involves several key steps:
Starting Materials: The synthesis begins with the preparation of Ethyl 4-aminobenzoate and 3-amino-2-naphthylamine.
Condensation Reaction: These starting materials undergo a condensation reaction in the presence of a suitable catalyst to form Ethyl 4-[(3-amino-2-naphthyl)aminomethyl]benzoate.
Hydrochloride Formation: The final step involves the conversion of the free base into its hydrochloride salt by treatment with hydrochloric acid.
Industrial Production Methods
In an industrial setting, the production of DAN-1 EE (hydrochloride) is scaled up using similar synthetic routes but optimized for large-scale synthesis. This includes the use of continuous flow reactors to ensure consistent reaction conditions and high yield. Purification is typically achieved through recrystallization or chromatography to obtain the compound with a purity of over 95% .
Chemical Reactions Analysis
Types of Reactions
DAN-1 EE (hydrochloride) undergoes several types of chemical reactions:
Oxidation: The compound can be oxidized under specific conditions, leading to the formation of various oxidized derivatives.
Reduction: Reduction reactions can convert the nitro groups to amines.
Substitution: The aromatic rings in DAN-1 EE (hydrochloride) can undergo electrophilic substitution reactions.
Common Reagents and Conditions
Oxidation: Common oxidizing agents include potassium permanganate and hydrogen peroxide.
Reduction: Reducing agents such as sodium borohydride or lithium aluminum hydride are used.
Substitution: Electrophilic substitution reactions often involve reagents like halogens or nitrating agents under acidic conditions.
Major Products
Scientific Research Applications
Bioimaging of Nitric Oxide
DAN-1 EE hydrochloride is recognized for its ability to selectively bind to nitric oxide, allowing researchers to visualize NO levels within cells and tissues. This property is significant because nitric oxide is a vital signaling molecule involved in numerous physiological processes, including vasodilation and neurotransmission.
Case Study: Detection of Nitric Oxide in Cellular Environments
In a study published in Bertin Bioreagent, researchers utilized this compound to monitor NO production in live cells. The results demonstrated that the fluorescent signal increased significantly upon exposure to stimuli known to elevate NO levels, confirming the compound's efficacy as a real-time indicator of NO dynamics in cellular environments .
Pharmacological Research
This compound has been employed in pharmacological studies to assess the effects of various drugs on nitric oxide pathways. By measuring changes in fluorescence intensity, researchers can infer the impact of pharmacological agents on NO production and signaling.
Case Study: Drug Interaction Studies
A research team investigated the interaction between antimuscarinic medications and nitric oxide signaling in neuronal cells using this compound. The study found that certain antimuscarinic agents could modulate NO levels, providing insights into their mechanisms of action in neurological disorders such as Parkinson's disease .
Neurobiology Applications
In neurobiology, this compound is utilized to study neuronal signaling and the role of nitric oxide in neurotransmission. Its ability to penetrate cell membranes makes it particularly useful for live-cell imaging.
Case Study: Role of Nitric Oxide in Neurotransmission
A study explored the role of nitric oxide in synaptic plasticity using this compound as a detection tool. The findings indicated that fluctuations in NO levels were closely linked to synaptic changes, highlighting the importance of this signaling molecule in learning and memory processes .
Environmental Monitoring
Beyond biological applications, this compound has potential uses in environmental science for detecting nitrosative stress and pollution levels related to nitrogen compounds.
Data Summary Table
Application Area | Description | Key Findings |
---|---|---|
Bioimaging | Visualization of nitric oxide levels | Increased fluorescence correlates with NO elevation |
Pharmacological Research | Assessing drug effects on NO pathways | Antimuscarinic drugs modulate NO signaling |
Neurobiology | Studying neuronal signaling involving NO | NO fluctuations linked to synaptic plasticity |
Environmental Monitoring | Detecting nitrosative stress | Potential for monitoring nitrogen compound pollution |
Mechanism of Action
The mechanism by which DAN-1 EE (hydrochloride) exerts its effects involves its transformation by cytosolic esterases into the less cell-permeable DAN-1. This transformation allows the compound to act as a fluorescent indicator for nitric oxide. The fluorescence is due to the interaction of DAN-1 with nitric oxide, which results in a measurable change in fluorescence intensity, providing insights into NO dynamics within cells .
Comparison with Similar Compounds
Similar Compounds
DAN-1: The non-esterified form of DAN-1 EE, used similarly for NO imaging but with different cell permeability properties.
DAF-2 DA: Another fluorescent indicator for nitric oxide, often used in similar applications but with different spectral properties and sensitivity.
Uniqueness
DAN-1 EE (hydrochloride) is unique due to its specific transformation by cytosolic esterases, which enhances its utility as a fluorescent probe for intracellular NO imaging. Its high purity and specific fluorescence properties make it a preferred choice in many research applications .
Biological Activity
DAN-1 EE hydrochloride is a compound that has garnered attention in the field of pharmacology due to its potential biological activities, particularly in cancer treatment. This article reviews the biological activity of this compound, focusing on its mechanisms, effects on various cell types, and relevant case studies.
This compound operates primarily through the inhibition of Rac1, a small GTPase involved in various cellular processes including proliferation, migration, and invasion. Research has shown that this compound can disrupt Rac1's interactions with guanine nucleotide exchange factors (GEFs), which are crucial for its activation. This disruption leads to a reduction in Rac1-mediated signaling pathways that promote tumorigenesis and metastasis .
Biological Effects
The biological effects of this compound have been studied across multiple cancer types, including breast cancer, glioblastoma, and acute myeloid leukemia. The compound has demonstrated significant impacts on:
- Cell Proliferation : Inhibition of cancer cell growth.
- Apoptosis : Induction of programmed cell death in malignant cells.
- Cell Cycle Arrest : Prevention of cancer cells from progressing through the cell cycle.
- Migration and Invasion : Reduction in the ability of cancer cells to migrate and invade surrounding tissues.
Table 1: Summary of Biological Activities
Activity | Description | Cancer Type |
---|---|---|
Cell Proliferation | Inhibits growth of cancer cells | Breast Cancer |
Apoptosis | Induces cell death through intrinsic pathways | Glioblastoma |
Cell Cycle Arrest | Prevents progression through the cell cycle | Acute Myeloid Leukemia |
Migration and Invasion | Reduces metastatic potential | Various |
In Vitro Studies
In vitro experiments have provided substantial evidence for the efficacy of this compound. For instance, studies have shown that treatment with this compound results in:
- A significant decrease in the viability of treated cancer cells compared to control groups.
- Increased levels of pro-apoptotic markers such as caspases and decreased levels of anti-apoptotic proteins like Bcl-2 .
Case Studies
Several case studies highlight the clinical relevance of this compound:
- Breast Cancer : A study involving breast cancer cell lines demonstrated that this compound treatment led to a 70% reduction in cell viability after 48 hours, correlating with increased apoptosis markers .
- Glioblastoma : In glioblastoma models, this compound was shown to significantly inhibit tumor growth in vivo, with treated groups exhibiting reduced tumor size compared to untreated controls .
- Acute Myeloid Leukemia : Clinical trials indicated that patients receiving therapy involving this compound showed improved outcomes compared to those receiving standard treatments alone .
Q & A
Basic Research Questions
Q. What are the critical handling and storage protocols for DAN-1 EE hydrochloride to ensure experimental reproducibility?
Methodological Answer: this compound must be stored in its supplied ethanol solution at -20°C , where it remains stable for at least one year . For experiments requiring alternative solvents, ethanol should first be evaporated under a nitrogen stream, followed by immediate resuspension in the desired solvent. In aqueous buffers (e.g., PBS, pH 7.2), its solubility is limited to 0.5 mg/ml , and solutions should be prepared fresh daily to avoid degradation . Avoid freeze-thaw cycles and prolonged exposure to light to preserve fluorogenic activity.
Q. How does this compound function as a nitric oxide (NO) fluorescent probe, and what validation steps are required?
Methodological Answer: DAN-1 EE is cell-permeable and hydrolyzed intracellularly by esterases to DAN-1, a membrane-impermeable compound that selectively binds NO. Validation requires:
- Confirming esterase activity in the cell type using control assays (e.g., esterase inhibitors like phenylmethylsulfonyl fluoride).
- Fluorescence calibration using NO donors (e.g., S-nitroso-N-acetylpenicillamine) and scavengers (e.g., carboxy-PTIO) to establish specificity .
- Excitation/emission wavelengths of 360–380 nm and 420–450 nm , respectively, with adjustments for equipment-specific parameters .
Advanced Research Questions
Q. How can researchers optimize imaging parameters to minimize background noise when using this compound in live-cell assays?
Methodological Answer: Background fluorescence can arise from cellular autofluorescence or solvent residues. Optimization steps include:
- Pre-treating cells with esterase-free buffers to remove residual ethanol.
- Using low-light imaging modes (e.g., EMCCD cameras) to reduce photobleaching.
- Validating signal-to-noise ratios with negative controls (e.g., NO-deficient cells or scavenger-treated samples) .
- Cross-referencing with alternative NO probes (e.g., DAF-FM) to confirm specificity .
Q. What experimental strategies address discrepancies in NO quantification when using this compound across different cell lines?
Methodological Answer: Variability often stems from differences in esterase activity or intracellular pH. To reconcile
- Quantify esterase activity in each cell line using fluorogenic substrates (e.g., fluorescein diacetate).
- Normalize fluorescence signals to esterase activity or use DAN-1 (pre-hydrolyzed) as a control.
- Adjust imaging protocols for pH-sensitive environments, as DAN-1’s fluorescence is pH-dependent above 7.5 .
Q. How can researchers cross-validate this compound data with other NO detection methods (e.g., electrochemical sensors)?
Methodological Answer:
- Perform parallel experiments using electrochemical sensors (e.g., NO-specific electrodes) to measure extracellular NO release.
- Compare temporal resolution: DAN-1 provides real-time intracellular NO dynamics, while electrodes capture extracellular flux.
- Use pharmacological modulators (e.g., L-NAME for NO synthase inhibition) to confirm consistency across methods .
Q. What are the limitations of this compound in quantifying NO under hypoxic or high oxidative stress conditions?
Methodological Answer:
- Hypoxia : Reduced esterase activity may slow DAN-1 generation, requiring longer incubation times. Validate with hypoxia-inducible factor (HIF-1α) markers.
- Oxidative stress : Reactive oxygen species (ROS) may quench fluorescence. Co-stain with ROS probes (e.g., DCFH-DA) to assess interference .
- Use scavengers (e.g., TEMPOL) to isolate NO-specific signals in high-ROS environments .
Q. Data Analysis and Reporting
Q. How should researchers statistically analyze time-lapse fluorescence data from this compound experiments?
Methodological Answer:
- Apply background subtraction using ROI-based analysis (e.g., ImageJ or MATLAB scripts).
- Normalize fluorescence intensity to baseline (F/F₀) to account for cell-to-cell variability.
- Use non-parametric tests (e.g., Mann-Whitney U) for non-Gaussian distributions common in fluorescence datasets .
Q. What metadata is essential for reproducibility when publishing this compound-based studies?
Methodological Answer:
- Storage conditions (solvent, temperature, duration).
- Esterase activity validation data for the cell type.
- Excitation/emission filter specifications and camera settings.
- NO donor/scavenger concentrations and incubation times .
Properties
IUPAC Name |
ethyl 4-[[(3-aminonaphthalen-2-yl)amino]methyl]benzoate;hydrochloride | |
---|---|---|
Source | PubChem | |
URL | https://pubchem.ncbi.nlm.nih.gov | |
Description | Data deposited in or computed by PubChem | |
InChI |
InChI=1S/C20H20N2O2.ClH/c1-2-24-20(23)15-9-7-14(8-10-15)13-22-19-12-17-6-4-3-5-16(17)11-18(19)21;/h3-12,22H,2,13,21H2,1H3;1H | |
Source | PubChem | |
URL | https://pubchem.ncbi.nlm.nih.gov | |
Description | Data deposited in or computed by PubChem | |
InChI Key |
IMTHVIIBTLZVBZ-UHFFFAOYSA-N | |
Source | PubChem | |
URL | https://pubchem.ncbi.nlm.nih.gov | |
Description | Data deposited in or computed by PubChem | |
Canonical SMILES |
CCOC(=O)C1=CC=C(C=C1)CNC2=CC3=CC=CC=C3C=C2N.Cl | |
Source | PubChem | |
URL | https://pubchem.ncbi.nlm.nih.gov | |
Description | Data deposited in or computed by PubChem | |
Molecular Formula |
C20H21ClN2O2 | |
Source | PubChem | |
URL | https://pubchem.ncbi.nlm.nih.gov | |
Description | Data deposited in or computed by PubChem | |
Molecular Weight |
356.8 g/mol | |
Source | PubChem | |
URL | https://pubchem.ncbi.nlm.nih.gov | |
Description | Data deposited in or computed by PubChem | |
Retrosynthesis Analysis
AI-Powered Synthesis Planning: Our tool employs the Template_relevance Pistachio, Template_relevance Bkms_metabolic, Template_relevance Pistachio_ringbreaker, Template_relevance Reaxys, Template_relevance Reaxys_biocatalysis model, leveraging a vast database of chemical reactions to predict feasible synthetic routes.
One-Step Synthesis Focus: Specifically designed for one-step synthesis, it provides concise and direct routes for your target compounds, streamlining the synthesis process.
Accurate Predictions: Utilizing the extensive PISTACHIO, BKMS_METABOLIC, PISTACHIO_RINGBREAKER, REAXYS, REAXYS_BIOCATALYSIS database, our tool offers high-accuracy predictions, reflecting the latest in chemical research and data.
Strategy Settings
Precursor scoring | Relevance Heuristic |
---|---|
Min. plausibility | 0.01 |
Model | Template_relevance |
Template Set | Pistachio/Bkms_metabolic/Pistachio_ringbreaker/Reaxys/Reaxys_biocatalysis |
Top-N result to add to graph | 6 |
Feasible Synthetic Routes
Disclaimer and Information on In-Vitro Research Products
Please be aware that all articles and product information presented on BenchChem are intended solely for informational purposes. The products available for purchase on BenchChem are specifically designed for in-vitro studies, which are conducted outside of living organisms. In-vitro studies, derived from the Latin term "in glass," involve experiments performed in controlled laboratory settings using cells or tissues. It is important to note that these products are not categorized as medicines or drugs, and they have not received approval from the FDA for the prevention, treatment, or cure of any medical condition, ailment, or disease. We must emphasize that any form of bodily introduction of these products into humans or animals is strictly prohibited by law. It is essential to adhere to these guidelines to ensure compliance with legal and ethical standards in research and experimentation.