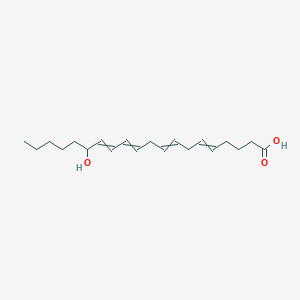
15(R)-Hete
Overview
Description
15(R)-Hydroxyeicosatetraenoic acid [15(R)-HETE] is a chiral enantiomer of 15(S)-HETE, a hydroxylated derivative of arachidonic acid (AA) produced via lipoxygenase (LOX) or cyclooxygenase (COX) pathways. Unlike 15(S)-HETE, which is primarily synthesized by 15-LOX-1/2 or COX-1, this compound is generated non-enzymatically through auto-oxidation of AA or via aspirin-acetylated COX-2 . Its stereochemical configuration (R vs. S) significantly impacts its biological activity, metabolic fate, and receptor interactions. For instance, this compound serves as a precursor for anti-inflammatory 15-epi-lipoxins (aspirin-triggered lipoxins) via transcellular metabolism with leukocyte 5-LOX .
Preparation Methods
Biosynthetic Preparation of 15(R)-HETE
Platelet-Mediated Biosynthesis
Platelets are a primary natural source of this compound. These cells metabolize arachidonic acid (AA) via 12-lipoxygenase (12-LO), which typically produces 12-hydroxyeicosatetraenoic acid (12-HETE). However, under specific conditions, platelets generate 15(R)-hydroperoxyeicosatetraenoic acid (15(R)-HpETE), which is rapidly reduced to this compound by cellular peroxidases . The process involves:
-
Isolation of Platelets : Human or bovine platelets are isolated via centrifugation from fresh blood samples.
-
Stimulation with Agonists : Platelets are activated using thrombin or collagen to trigger AA release from membrane phospholipids.
-
Incubation with AA : Exogenous AA is added to the platelet suspension, and the mixture is incubated at 37°C under controlled oxygen levels.
-
Enzymatic Reduction : 15(R)-HpETE is reduced to this compound by glutathione peroxidase or other cellular reductases .
This method yields this compound in nanomolar concentrations, necessitating purification via high-performance liquid chromatography (HPLC) .
Hypoxia-Induced Microsomal Synthesis
Chronic hypoxia activates 15-lipoxygenase (15-LO) in lung tissues, leading to 15-HETE synthesis. While this pathway predominantly produces the S-isomer, stereochemical inversion can occur under specific conditions . Key steps include:
-
Microsome Preparation : Lung tissues from hypoxia-exposed rabbits are homogenized, and microsomes are isolated via differential centrifugation.
-
Enzymatic Incubation : Microsomes are incubated with AA (9–14 μM) in oxygenated buffer (pH 7.4) at 37°C.
-
Inhibition of Competing Pathways : Cytochrome P450 and cyclooxygenase inhibitors (e.g., 17-ODYA, indomethacin) are added to suppress alternative AA metabolic routes .
Gas chromatography/mass spectrometry (GC/MS) confirms the identity of 15-HETE, though distinguishing R/S isomers requires chiral chromatography .
Chemical Synthesis and Auto-Oxidation
Asymmetric Chemical Synthesis
Chemical synthesis of this compound involves stereoselective oxidation of AA. A representative approach includes:
-
Chiral Epoxidation : AA is treated with a Sharpless asymmetric epoxidation catalyst to introduce an epoxy group at C14–C15.
-
Epoxide Opening : The epoxy intermediate is hydrolyzed using a chiral Lewis acid to yield this compound with >90% enantiomeric excess (ee) .
This method achieves milligram-scale production but requires advanced chiral separation techniques, such as normal-phase HPLC with silica columns .
Auto-Oxidation Pathways
Non-enzymatic auto-oxidation of AA generates racemic 15-HETE through free radical-mediated peroxidation. The R-isomer can be isolated via:
-
Controlled Oxidation : AA is incubated with Fe²⁺/ascorbate in phosphate buffer (pH 7.4) at 37°C for 24 hours.
-
Chiral Resolution : The racemic mixture is separated using a Chiralpak AD-H column with hexane/isopropanol/acetic acid (96:4:0.1) mobile phase .
Auto-oxidation yields sub-milligram quantities of this compound, limiting its utility for large-scale applications .
Analytical Validation of this compound
High-Performance Liquid Chromatography (HPLC)
Reverse-phase HPLC (rp-HPLC) and normal-phase HPLC (np-HPLC) are critical for purifying and resolving this compound:
-
rp-HPLC : A C18 column with acetonitrile/water (0.1% acetic acid) gradient elution resolves 15-HETE from other eicosanoids .
-
np-HPLC : A silica column with hexane/isopropanol/acetic acid (98:2:0.1) separates R and S isomers .
Gas Chromatography/Mass Spectrometry (GC/MS)
GC/MS validates structural integrity and stereochemistry:
-
Derivatization : this compound is converted to its methyl ester trimethylsilyl (TMS) ether derivative.
-
Fragmentation Analysis : Characteristic ions (e.g., m/z 391 [M-TMSOH]⁺) confirm the hydroxy group position and configuration .
Comparative Efficiency of Preparation Methods
Method | Yield (mg/L) | Enantiomeric Excess (%) | Key Advantages | Limitations |
---|---|---|---|---|
Platelet Biosynthesis | 0.05–0.1 | 95–98 | Biologically relevant, high specificity | Low yield, complex purification |
Microsomal Synthesis | 0.1–0.3 | 90–95 | Scalable, enzyme-driven | Requires hypoxia models |
Chemical Synthesis | 10–50 | >90 | High purity, customizable | Costly reagents, technical expertise |
Auto-Oxidation | 0.5–1.0 | 50–60 | Simple, no enzymes required | Racemic mixture, low ee |
Chemical Reactions Analysis
Metabolic Pathways and Conversions
-
Oxidation to 15-oxo-ETE: 15(R)-HETE can be oxidized by NAD-dependent 5-hydroxyprostaglandin dehydrogenase to form 15-oxo-ETE .
-
Lipoxin Formation: 15(R)-HETE can be oxygenated by 5-lipoxygenase (ALOX5) to form its 5,6-oxido derivative, which rearranges to form the 15(R) diastereomers of lipoxin A4 (LXA4) and lipoxin B4 (LXB4), namely 15-epi-LXA4 and 15-epi-LXB4 .
-
Incorporation into Phospholipids: Similar to 15(S)-HpETE, 15(R)-HETE can be acylated into membrane phospholipids .
-
Further Metabolism by Cytochrome P450 Enzymes: Cytochrome P450 enzymes can further metabolize 15(R)-HETE, leading to various products .
Key Chemical Reactions of this compound
Reaction Type | Reactant(s) | Enzyme/Catalyst | Product(s) |
---|---|---|---|
Oxidation | 15(R)-HETE | NAD-dependent 5-hydroxyprostaglandin dehydrogenase | 15-oxo-ETE |
Oxygenation | 15(R)-HETE | ALOX5 | 5,6-oxido derivative of 15(R)-HETE → 15-epi-LXA4 and 15-epi-LXB4 |
Reduction | 15(R)-HpETE | Cellular peroxidases | 15(R)-HETE |
Formation from Arachidonic Acid | Arachidonic Acid | Microsomal Cytochrome P450s (e.g., CYP2C19) | Racemic mixture of 15-HETEs, >90% as 15(R) stereoisomer |
Autoxidation | Arachidonic Acid (Oxidative Stress) | Non-enzymatic | 15(R,S)-hydroperoxy-5Z,8Z,11Z,13E-eicosatetraenoic acids → 15(R,S)-HETEs and other products |
Biological Activities and Significance
-
Peroxisome Proliferator-Activated Receptor (PPAR) Activation: 15-HETE, including both 15(R)-HETE and 15(S)-HETE enantiomers, can activate PPARs . They act as agonists for PPARbeta/delta, PPARalpha, and PPARgamma, influencing target gene expression .
-
Role in Angiogenesis and Functional Recovery: 15-HETE is involved in angiogenesis and neurological functional recovery after cerebral ischemia . It stimulates the proliferation and migration of brain microvascular endothelial cells (BMVECs) .
-
Involvement in Pathological Conditions: 15-HETE is implicated in various pathological conditions, including cancer, hypertension, allergic rhinitis, and arthritis .
-
Pulmonary Hypertension: A diet rich in 15-HETE can induce pulmonary hypertension through a cytotoxic T cell-dependent mechanism .
-
Allergic Reactions: 15-HETE, generated by basophils, may mediate allergic reactions .
Detection and Measurement
-
ELISA Kits: ELISA (Enzyme-Linked Immunosorbent Assay) kits are available for quantifying 15-HETE levels in biological samples . These kits use antibodies against 15-HETE and can be employed for measuring 15-LOX activity .
Implications in Disease
-
Cancer: 15(S)-HETE and its forming enzyme, 15-LOX-2, may act as tumor suppressors. A decline in 15-HETE production in cancer cells may help them avoid apoptosis .
-
Inflammation and Allergy: 15-HETE is associated with IgE-mediated allergic reactions and may play a role in immediate allergenic responses .
-
Cardiovascular Diseases: 15-HETE is implicated in hypertension and pulmonary hypertension . It affects pulmonary artery endothelial cells (PAECs) and can induce pulmonary vascular wall thickening .
Scientific Research Applications
Role in Inflammation and Allergic Reactions
15(R)-HETE has been implicated in various inflammatory processes. Research suggests that this compound plays a crucial role in mediating allergic reactions. For instance, studies have shown that 15-HETE levels increase significantly in leukocytes from allergic patients upon allergen exposure. The generation of 15-HETE correlates with histamine release, indicating its potential as a mediator in immediate allergic responses .
Angiogenesis and Ischemic Stroke Recovery
A key application of this compound is its involvement in angiogenesis, particularly in the context of ischemic stroke. The 15-LO/15-HETE signaling pathway has been shown to promote the expression of vascular endothelial growth factor (VEGF), which is essential for new blood vessel formation. In animal models of cerebral ischemia, administration of 15-HETE enhanced microvascular density and improved neurological outcomes following stroke .
Case Study: Cerebral Ischemia Model
- Model : Mouse model of middle cerebral artery occlusion (MCAO).
- Findings : Increased expression of VEGF and enhanced angiogenesis were observed after treatment with 15-HETE.
- Outcome : Improved functional recovery was noted, with enhanced performance in motor tests post-stroke .
Influence on Asthma Pathophysiology
In respiratory conditions such as asthma, this compound has been identified as a significant metabolite influencing airway inflammation. It is the most abundant arachidonic acid metabolite found in the human lung, suggesting its role in modulating bronchial responses. Studies indicate that mast cells, which are pivotal in asthma pathophysiology, may produce 15-HETE through both COX and lipoxygenase pathways .
Potential Therapeutic Applications
Given its roles in inflammation and tissue repair processes, this compound is being explored for therapeutic applications:
- Anti-inflammatory therapies : Due to its ability to modulate inflammatory responses, this compound analogs are being investigated as potential treatments for chronic inflammatory diseases.
- Neuroprotective strategies : The enhancement of angiogenesis and neurobehavioral recovery post-stroke positions this compound as a candidate for therapeutic interventions aimed at improving outcomes after cerebral ischemia .
Summary Table of Applications
Mechanism of Action
15(S)-HETE exerts its effects through various molecular targets and pathways. It binds to specific receptors on the cell surface, activating signaling pathways that regulate gene expression, cell proliferation, and apoptosis. One of the key pathways involves the activation of Src kinase, which leads to the induction of early growth response protein 1 (Egr-1) and subsequent expression of FGF-2 . Additionally, 15(S)-HETE increases intracellular calcium levels, contributing to its vasoconstrictive and pro-inflammatory effects .
Comparison with Similar Compounds
15(S)-HETE
- Structural Similarity: Both are hydroxylated eicosanoids with identical molecular formulas (C20H32O3) but differ in stereochemistry at the C15 position.
- Biosynthesis: 15(S)-HETE: Enzymatically produced by 15-LOX-1/2 or COX-1 . 15(R)-HETE: Generated non-enzymatically or via aspirin-acetylated COX-2 .
- Biological Activity: Vasoconstriction: 15(S)-HETE exhibits potent vasoconstrictive effects in rabbit pulmonary arteries (EC50: ~5.6 μM), while this compound shows negligible activity . Anti-inflammatory Pathways: 15(S)-HETE is metabolized to pro-resolving 15-oxo-ETE, while this compound is converted to 15-epi-lipoxins, which have enhanced anti-inflammatory properties .
- Metabolic Stability : this compound is more stable than 15(S)-HETE under oxidative conditions (94% decomposition at 75°C after 330 hours vs. 78% for 15(S)-HETE at 0.25 hours) .
15-oxo-ETE
- Relationship : 15-oxo-ETE is a downstream metabolite of 15(S)-HETE, produced via 15-hydroxyprostaglandin dehydrogenase (15-PGDH) .
- Function: 15-oxo-ETE exhibits chemotactic and pro-angiogenic effects, contrasting with the anti-inflammatory role of this compound-derived metabolites .
11(R)-HETE
- Structural Comparison: Both 11(R)-HETE and this compound are R-configuration eicosanoids, but they differ in hydroxylation sites (C11 vs. C15).
- Biosynthetic Pathways :
- Biological Roles :
Data Tables
Table 2. Enzymatic Sources and Key Metabolites
Compound | Primary Enzymatic Source | Key Metabolites | Biological Role |
---|---|---|---|
15(S)-HETE | 15-LOX-1, COX-1 | 15-oxo-ETE | Pro-angiogenic, vasoconstrictive |
This compound | Aspirin-COX-2, auto-oxidation | 15-epi-lipoxins | Anti-inflammatory |
11(R)-HETE | COX-1/2 | 11-oxo-ETE | Weak PPARγ activation |
Research Findings and Implications
- Stereochemical Impact : The R-configuration of 15-HETE reduces its potency in vasoconstriction and cell proliferation but enhances its role in anti-inflammatory signaling .
- Therapeutic Potential: this compound-derived 15-epi-lipoxins are promising candidates for treating inflammatory diseases, while 15(S)-HETE inhibitors may mitigate cardiovascular pathologies .
- Technical Challenges: Differentiating 15(R)- and 15(S)-HETE in assays requires chiral chromatography or discriminatory immunoassays due to their structural similarity .
Biological Activity
15(R)-Hydroxyeicosatetraenoic acid (15(R)-HETE) is a biologically active lipid mediator derived from arachidonic acid. It plays a significant role in various physiological and pathological processes, including inflammation, vascular function, and cell proliferation. This article reviews the biological activity of this compound, focusing on its mechanisms of action, effects on different cell types, and implications in health and disease.
15-HETE is produced through the metabolism of arachidonic acid by lipoxygenases (LOXs) and cyclooxygenases (COXs). Specifically, this compound is primarily synthesized via COX-1 activity in human mast cells, where it has been shown to accumulate during physiological conditions while the 15(S) isomer is metabolized further by dehydrogenases .
Table 1: Biosynthetic Pathways of 15-HETE
Enzyme | Substrate | Product | Notes |
---|---|---|---|
15-LOX | Arachidonic Acid | 15(S)-HpETE | Short-lived hydroperoxide |
COX-1 | Arachidonic Acid | This compound | Predominantly produced in human mast cells |
15-Hydroxy PGD | 15(S)-HpETE | 15(S)-HETE | Further metabolized to various derivatives |
Vascular Effects
This compound exhibits potent vasoconstrictive properties. Studies have shown that it can induce concentration-dependent contractions in isolated pulmonary arteries from rabbits, with effects being more pronounced in female subjects compared to males . This sex-specific response suggests that hormonal differences may play a role in the vascular reactivity to this eicosanoid.
Proliferative Effects
The compound has been implicated in cell proliferation, particularly in vascular smooth muscle cells. Research indicates that both this compound and its isomer, 15(S)-HETE, enhance thymidine incorporation into these cells, suggesting a mitogenic effect . However, contrasting studies have reported that while it promotes proliferation in some contexts, it can inhibit growth in cancer cell lines such as PC-3 prostate carcinoma cells .
Inflammatory Response
This compound is involved in inflammatory processes. It acts as an autocrine and paracrine signaling molecule that can modulate immune responses. For instance, it has been shown to influence the activity of platelets and other immune cells during inflammation . The compound's role as a precursor to other bioactive lipids further underscores its significance in mediating inflammatory responses.
Case Studies
- Asthma Pathophysiology : In studies examining human mast cells, researchers found that 15-HETE levels correlate with asthma severity. The predominant production of the 15(R) isomer suggests a potential biomarker for assessing asthma-related inflammation .
- Cardiovascular Health : Elevated levels of 15-HETE have been associated with hypertension and other cardiovascular diseases. Its ability to stimulate vascular smooth muscle proliferation indicates a potential role in atherogenesis and vascular remodeling .
Q & A
Basic Research Questions
Q. What are the established biosynthetic pathways for 15(R)-HETE, and how do they differ from its enantiomer 15(S)-HETE?
- Methodological Answer : Biosynthesis can be studied using stable isotope-labeled arachidonic acid precursors in cell cultures (e.g., endothelial cells or leukocytes). Liquid chromatography-tandem mass spectrometry (LC-MS/MS) is critical for distinguishing this compound from 15(S)-HETE due to their stereochemical differences. Enzymatic assays with cyclooxygenase (COX) or lipoxygenase (LOX) isoforms, particularly 15-LOX-2, should be prioritized to clarify pathway specificity .
Q. How can researchers reliably detect and quantify this compound in biological samples?
- Methodological Answer : Use chiral chromatography coupled with MS/MS to resolve enantiomers. Validate assays with synthetic standards for both 15(R)- and 15(S)-HETE. Include internal standards (e.g., deuterated analogs) to correct for matrix effects. Protocols should adhere to guidelines for lipid mediator analysis, as outlined in Reviews in Analytical Chemistry .
Q. What receptor systems are activated by this compound, and how do these interactions compare to other eicosanoids?
- Methodological Answer : Employ competitive binding assays (e.g., fluorescence polarization) and reporter gene systems (e.g., PPAR-responsive luciferase constructs) to assess receptor activation. Compare this compound’s affinity for PPAR isoforms (α, β/δ, γ) against other HETEs and prostaglandins. Note that this compound exhibits weaker PPARγ activation than 15(S)-HETE, as shown in macrophage studies .
Advanced Research Questions
Q. How should experimental designs address contradictory data on this compound’s role in inflammatory vs. anti-inflammatory processes?
- Methodological Answer : Conduct context-specific studies (e.g., cell type, disease model) with rigorous controls. For example, in atherosclerosis models, compare this compound’s effects on foam cell formation (via CD36 expression) against its enantiomer. Use RNA interference (siRNA/ASO) to isolate pathways (e.g., ROS/Syk/Pyk2) and resolve mechanistic contradictions . Meta-analyses of existing datasets, pre-registered on platforms like PROSPERO, can identify confounding variables .
Q. What strategies ensure reproducibility when comparing this compound’s enantiomer-specific effects in vivo?
- Methodological Answer : Use stereochemically pure compounds validated by nuclear magnetic resonance (NMR). In animal models (e.g., ApoE⁻/⁻ mice for atherosclerosis), administer enantiomers via controlled delivery systems (e.g., osmotic pumps). Blind experiments and predefine endpoints (e.g., plaque size, cytokine levels) to minimize bias. Report adherence to ARRIVE guidelines .
Q. How can researchers resolve discrepancies in this compound’s metabolic fate across different tissues?
- Methodological Answer : Apply tracer-based metabolomics with ¹⁴C- or ¹³C-labeled this compound in tissue explants (e.g., lung vs. liver). Combine with CRISPR-Cas9 knockout models to identify enzymes responsible for its conversion (e.g., cytochrome P450s). Data should be analyzed using pathway enrichment tools (e.g., MetaboAnalyst) .
Q. What computational models predict this compound’s interactions with lipid-binding proteins?
- Methodological Answer : Use molecular docking (e.g., AutoDock Vina) and molecular dynamics simulations (e.g., GROMACS) to model this compound’s binding to CD36 or albumin. Validate predictions with surface plasmon resonance (SPR) to measure binding kinetics. Cross-reference with crystallographic data from related eicosanoid-protein complexes .
Q. How do experimental conditions (e.g., oxygen tension) influence this compound synthesis and stability?
- Methodological Answer : Perform hypoxia-reoxygenation experiments in controlled bioreactors. Quantify this compound using LC-MS/MS under varying O₂ levels (5–21%). Assess stability via accelerated degradation studies (e.g., pH/temperature stress) with UPLC-UV monitoring. Statistical models (e.g., ANOVA with Tukey’s post-hoc) should identify significant environmental interactions .
Q. Data Analysis and Interpretation
Q. What statistical approaches are optimal for analyzing dose-response relationships in this compound studies?
- Methodological Answer : Use nonlinear regression (e.g., four-parameter logistic model) to calculate EC₅₀ values. For RNA-seq data on CD36 expression, apply DESeq2 or edgeR for differential analysis. Sensitivity analyses (e.g., Monte Carlo simulations) can address variability in biological replicates .
Q. How should researchers handle missing data in longitudinal studies of this compound’s metabolic effects?
- Methodological Answer : Implement multiple imputation (MI) with chained equations (MICE) or maximum likelihood estimation. Validate imputations using Rubin’s rules. Pre-register data exclusion criteria to avoid post-hoc bias .
Q. Ethical and Reporting Standards
Q. What transparency measures are critical for HETE-related hypothesis-testing studies (HETE)?
- Methodological Answer : Pre-specify hypotheses, analysis plans, and endpoints in registries (e.g., ClinicalTrials.gov or Open Science Framework). For exploratory studies, clearly distinguish hypothesis-generating vs. confirmatory analyses. Follow CONSORT-EHEALTH guidelines for RCTs or STROBE for observational studies .
Q. How can authors ensure compliance with chemical data reporting standards in journals?
- Methodological Answer : Adhere to the Beilstein Journal of Organic Chemistry guidelines: report synthetic procedures for novel compounds, validate identity/purity via NMR and HRMS, and deposit raw data in repositories (e.g., Zenodo). Use IUPAC nomenclature and avoid nonstandard abbreviations .
Properties
IUPAC Name |
15-hydroxyicosa-5,8,11,13-tetraenoic acid | |
---|---|---|
Source | PubChem | |
URL | https://pubchem.ncbi.nlm.nih.gov | |
Description | Data deposited in or computed by PubChem | |
InChI |
InChI=1S/C20H32O3/c1-2-3-13-16-19(21)17-14-11-9-7-5-4-6-8-10-12-15-18-20(22)23/h4-5,8-11,14,17,19,21H,2-3,6-7,12-13,15-16,18H2,1H3,(H,22,23) | |
Source | PubChem | |
URL | https://pubchem.ncbi.nlm.nih.gov | |
Description | Data deposited in or computed by PubChem | |
InChI Key |
JSFATNQSLKRBCI-UHFFFAOYSA-N | |
Source | PubChem | |
URL | https://pubchem.ncbi.nlm.nih.gov | |
Description | Data deposited in or computed by PubChem | |
Canonical SMILES |
CCCCCC(C=CC=CCC=CCC=CCCCC(=O)O)O | |
Source | PubChem | |
URL | https://pubchem.ncbi.nlm.nih.gov | |
Description | Data deposited in or computed by PubChem | |
Molecular Formula |
C20H32O3 | |
Source | PubChem | |
URL | https://pubchem.ncbi.nlm.nih.gov | |
Description | Data deposited in or computed by PubChem | |
DSSTOX Substance ID |
DTXSID20866448 | |
Record name | 15-Hydroxyicosa-5,8,11,13-tetraenoic acid | |
Source | EPA DSSTox | |
URL | https://comptox.epa.gov/dashboard/DTXSID20866448 | |
Description | DSSTox provides a high quality public chemistry resource for supporting improved predictive toxicology. | |
Molecular Weight |
320.5 g/mol | |
Source | PubChem | |
URL | https://pubchem.ncbi.nlm.nih.gov | |
Description | Data deposited in or computed by PubChem | |
CAS No. |
73945-47-8 | |
Record name | 15-Hydroxyicosa-5,8,11,13-tetraenoic acid | |
Source | EPA DSSTox | |
URL | https://comptox.epa.gov/dashboard/DTXSID20866448 | |
Description | DSSTox provides a high quality public chemistry resource for supporting improved predictive toxicology. | |
Retrosynthesis Analysis
AI-Powered Synthesis Planning: Our tool employs the Template_relevance Pistachio, Template_relevance Bkms_metabolic, Template_relevance Pistachio_ringbreaker, Template_relevance Reaxys, Template_relevance Reaxys_biocatalysis model, leveraging a vast database of chemical reactions to predict feasible synthetic routes.
One-Step Synthesis Focus: Specifically designed for one-step synthesis, it provides concise and direct routes for your target compounds, streamlining the synthesis process.
Accurate Predictions: Utilizing the extensive PISTACHIO, BKMS_METABOLIC, PISTACHIO_RINGBREAKER, REAXYS, REAXYS_BIOCATALYSIS database, our tool offers high-accuracy predictions, reflecting the latest in chemical research and data.
Strategy Settings
Precursor scoring | Relevance Heuristic |
---|---|
Min. plausibility | 0.01 |
Model | Template_relevance |
Template Set | Pistachio/Bkms_metabolic/Pistachio_ringbreaker/Reaxys/Reaxys_biocatalysis |
Top-N result to add to graph | 6 |
Feasible Synthetic Routes
Disclaimer and Information on In-Vitro Research Products
Please be aware that all articles and product information presented on BenchChem are intended solely for informational purposes. The products available for purchase on BenchChem are specifically designed for in-vitro studies, which are conducted outside of living organisms. In-vitro studies, derived from the Latin term "in glass," involve experiments performed in controlled laboratory settings using cells or tissues. It is important to note that these products are not categorized as medicines or drugs, and they have not received approval from the FDA for the prevention, treatment, or cure of any medical condition, ailment, or disease. We must emphasize that any form of bodily introduction of these products into humans or animals is strictly prohibited by law. It is essential to adhere to these guidelines to ensure compliance with legal and ethical standards in research and experimentation.