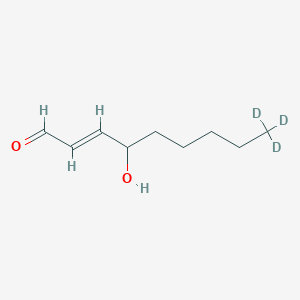
(E)-9,9,9-Trideuterio-4-hydroxynon-2-enal
Overview
Description
4-Hydroxy Nonenal-d3 is a deuterated form of 4-Hydroxy Nonenal, an α,β-unsaturated hydroxyalkenal. It is a lipid peroxidation product derived from the oxidation of polyunsaturated omega-6 fatty acids such as arachidonic acid and linoleic acid . This compound is widely used as an internal standard for the quantification of 4-Hydroxy Nonenal by gas chromatography or liquid chromatography-mass spectrometry . It is a colorless oil and is known for its various biological activities, including cytotoxicity, growth inhibition, genotoxicity, and chemotactic activity .
Mechanism of Action
Target of Action
4-Hydroxynonenal-d3, also known as 4-HNE-d3, is a deuterium-labeled variant of 4-Hydroxynonenal (4-HNE). The primary target of 4-HNE-d3 is acetaldehyde dehydrogenase 2 (ALDH2) . ALDH2 is an enzyme that plays a crucial role in alcohol metabolism by converting acetaldehyde, a toxic intermediate of alcohol metabolism, into non-toxic acetate .
Mode of Action
4-HNE-d3 interacts with its target, ALDH2, by acting as both a substrate and an inhibitor . It forms covalent adducts with nucleophilic functional groups in proteins, nucleic acids, and membrane lipids . This interaction primarily involves a Michael addition reaction, which can target cysteine, histidine, or lysine residues, or through the formation of a Schiff base, which can target arginine or lysine .
Biochemical Pathways
The action of 4-HNE-d3 affects various biochemical pathways. It is generated in the oxidation of lipids containing polyunsaturated omega-6 fatty acids, such as arachidonic and linoleic acids . The compound can modulate a number of signaling processes, primarily through the formation of covalent adducts with proteins, nucleic acids, and membrane lipids .
Pharmacokinetics
It is known that the compound is a product of lipid peroxidation, and its levels can increase during oxidative stress . The metabolism of 4-HNE-d3 leads to a great number of degradation products, some of which are excreted in urine and may serve as non-invasive biomarkers of oxidative stress .
Result of Action
The molecular and cellular effects of 4-HNE-d3’s action are diverse. It plays an important role in cancer through mitochondria . The compound’s ability to form adducts with proteins can lead to changes in protein function and cellular processes .
Action Environment
The action of 4-HNE-d3 can be influenced by various environmental factors. For instance, conditions that increase oxidative stress, such as exposure to toxins or radiation, can increase the production of 4-HNE-d3 . Furthermore, the compound’s reactivity and stability can be affected by factors such as pH and the presence of other reactive species .
Biochemical Analysis
Biochemical Properties
4-Hydroxynonenal-d3, like its non-deuterated counterpart, is known to interact with a variety of biomolecules. It can form covalent adducts with nucleophilic functional groups in proteins, nucleic acids, and membrane lipids . This interaction primarily occurs through a Michael addition reaction, targeting amino acids such as cysteine, histidine, or lysine . These biochemical reactions contribute to the role of 4-Hydroxynonenal-d3 in cellular signaling and stress responses .
Cellular Effects
4-Hydroxynonenal-d3 has been found to influence various cellular processes. It can modulate cell signaling pathways, gene expression, and cellular metabolism . For instance, it has been shown to inhibit the enzyme acetaldehyde dehydrogenase 2 (ALDH2), which is involved in alcohol metabolism . Furthermore, it has been implicated in the regulation of cell proliferation and transformation, and it can induce cell death under certain conditions .
Molecular Mechanism
The molecular mechanism of action of 4-Hydroxynonenal-d3 involves its binding interactions with biomolecules and subsequent changes in gene expression. It forms covalent adducts with proteins, nucleic acids, and membrane lipids, which can alter their function and disrupt normal cellular processes . For example, it can inhibit enzymes or activate them, leading to changes in metabolic pathways .
Temporal Effects in Laboratory Settings
The effects of 4-Hydroxynonenal-d3 can change over time in laboratory settings. It has been observed that the compound’s stability, degradation, and long-term effects on cellular function can vary in in vitro or in vivo studies
Metabolic Pathways
4-Hydroxynonenal-d3 is involved in the metabolic pathways of lipid peroxidation, specifically the oxidation of n-6 polyunsaturated fatty acids . It can interact with various enzymes and cofactors within these pathways. Specific details about these interactions and their effects on metabolic flux or metabolite levels are not currently available in the literature.
Transport and Distribution
It is known that 4-Hydroxynonenal-d3 can form adducts with proteins, nucleic acids, and membrane lipids, which could potentially influence its localization or accumulation . Specific details about transporters or binding proteins that it interacts with are not currently available in the literature.
Subcellular Localization
It is possible that it could be directed to specific compartments or organelles through targeting signals or post-translational modifications, given its ability to form adducts with various biomolecules . Specific details about these processes are not currently available in the literature.
Preparation Methods
Synthetic Routes and Reaction Conditions
4-Hydroxy Nonenal-d3 is synthesized by the oxidation of lipids containing polyunsaturated omega-6 fatty acids . The synthetic route involves the use of deuterated reagents to introduce deuterium atoms at specific positions in the molecule. The reaction conditions typically involve the use of oxidizing agents such as hydrogen peroxide or other peroxides under controlled temperature and pressure conditions .
Industrial Production Methods
Industrial production of 4-Hydroxy Nonenal-d3 involves large-scale lipid oxidation processes. The process starts with the extraction of polyunsaturated fatty acids from natural sources, followed by their oxidation in the presence of deuterated reagents. The product is then purified using techniques such as distillation or chromatography to obtain high-purity 4-Hydroxy Nonenal-d3 .
Chemical Reactions Analysis
Types of Reactions
4-Hydroxy Nonenal-d3 undergoes various types of chemical reactions, including:
Oxidation: The compound can be further oxidized to form various oxidation products.
Reduction: Reduction of the carbonyl group to an alcohol.
Substitution: The hydroxyl group can be substituted with other functional groups.
Michael Addition: The compound can undergo Michael addition reactions with nucleophiles such as thiols, amines, and other nucleophilic species.
Common Reagents and Conditions
Oxidizing Agents: Hydrogen peroxide, peracids.
Reducing Agents: Sodium borohydride, lithium aluminum hydride.
Nucleophiles: Thiols, amines, alcohols.
Major Products Formed
Oxidation Products: Various aldehydes and carboxylic acids.
Reduction Products: Alcohols.
Substitution Products: Compounds with substituted functional groups.
Michael Addition Products: Adducts with nucleophiles.
Scientific Research Applications
4-Hydroxy Nonenal-d3 is extensively used in scientific research due to its role as a marker of lipid peroxidation. Its applications include:
Comparison with Similar Compounds
4-Hydroxy Nonenal-d3 is unique due to the presence of deuterium atoms, which makes it an ideal internal standard for mass spectrometry analysis. Similar compounds include:
4-Hydroxy Nonenal: The non-deuterated form, which is also a lipid peroxidation product with similar biological activities.
4-Hydroxy-trans-2-hexenal: Another lipid peroxidation product derived from omega-3 fatty acids.
4-Hydroperoxy-trans-2-nonenal: An oxygenated α,β-unsaturated aldehyde generated from lipid oxidation.
4,5-Epoxy-trans-2-decenal: A related compound formed during lipid peroxidation.
These compounds share similar chemical properties and biological activities but differ in their specific molecular structures and the types of fatty acids from which they are derived.
Properties
IUPAC Name |
(E)-9,9,9-trideuterio-4-hydroxynon-2-enal | |
---|---|---|
Source | PubChem | |
URL | https://pubchem.ncbi.nlm.nih.gov | |
Description | Data deposited in or computed by PubChem | |
InChI |
InChI=1S/C9H16O2/c1-2-3-4-6-9(11)7-5-8-10/h5,7-9,11H,2-4,6H2,1H3/b7-5+/i1D3 | |
Source | PubChem | |
URL | https://pubchem.ncbi.nlm.nih.gov | |
Description | Data deposited in or computed by PubChem | |
InChI Key |
JVJFIQYAHPMBBX-PKJLGKQPSA-N | |
Source | PubChem | |
URL | https://pubchem.ncbi.nlm.nih.gov | |
Description | Data deposited in or computed by PubChem | |
Canonical SMILES |
CCCCCC(C=CC=O)O | |
Source | PubChem | |
URL | https://pubchem.ncbi.nlm.nih.gov | |
Description | Data deposited in or computed by PubChem | |
Isomeric SMILES |
[2H]C([2H])([2H])CCCCC(/C=C/C=O)O | |
Source | PubChem | |
URL | https://pubchem.ncbi.nlm.nih.gov | |
Description | Data deposited in or computed by PubChem | |
Molecular Formula |
C9H16O2 | |
Source | PubChem | |
URL | https://pubchem.ncbi.nlm.nih.gov | |
Description | Data deposited in or computed by PubChem | |
Molecular Weight |
159.24 g/mol | |
Source | PubChem | |
URL | https://pubchem.ncbi.nlm.nih.gov | |
Description | Data deposited in or computed by PubChem | |
Retrosynthesis Analysis
AI-Powered Synthesis Planning: Our tool employs the Template_relevance Pistachio, Template_relevance Bkms_metabolic, Template_relevance Pistachio_ringbreaker, Template_relevance Reaxys, Template_relevance Reaxys_biocatalysis model, leveraging a vast database of chemical reactions to predict feasible synthetic routes.
One-Step Synthesis Focus: Specifically designed for one-step synthesis, it provides concise and direct routes for your target compounds, streamlining the synthesis process.
Accurate Predictions: Utilizing the extensive PISTACHIO, BKMS_METABOLIC, PISTACHIO_RINGBREAKER, REAXYS, REAXYS_BIOCATALYSIS database, our tool offers high-accuracy predictions, reflecting the latest in chemical research and data.
Strategy Settings
Precursor scoring | Relevance Heuristic |
---|---|
Min. plausibility | 0.01 |
Model | Template_relevance |
Template Set | Pistachio/Bkms_metabolic/Pistachio_ringbreaker/Reaxys/Reaxys_biocatalysis |
Top-N result to add to graph | 6 |
Feasible Synthetic Routes
Disclaimer and Information on In-Vitro Research Products
Please be aware that all articles and product information presented on BenchChem are intended solely for informational purposes. The products available for purchase on BenchChem are specifically designed for in-vitro studies, which are conducted outside of living organisms. In-vitro studies, derived from the Latin term "in glass," involve experiments performed in controlled laboratory settings using cells or tissues. It is important to note that these products are not categorized as medicines or drugs, and they have not received approval from the FDA for the prevention, treatment, or cure of any medical condition, ailment, or disease. We must emphasize that any form of bodily introduction of these products into humans or animals is strictly prohibited by law. It is essential to adhere to these guidelines to ensure compliance with legal and ethical standards in research and experimentation.