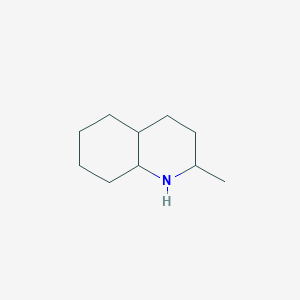
Decahydroquinoline, 2-methyl
Overview
Description
2-Methyldecahydroquinoline (C₁₀H₁₉N, molecular weight: 153.2646 g/mol) is a fully saturated bicyclic amine with a methyl substituent at the 2-position of the decahydroquinoline scaffold. Its stereoisomeric complexity arises from the fused cyclohexane and piperidine rings, which adopt distinct conformations depending on the hydrogenation pattern . The compound is characterized by its Kovats Retention Index (RI) of 1,190 (measured on an OV-101 non-polar column at 120°C, helium carrier gas), a critical parameter for chromatographic identification .
Preparation Methods
Catalytic Hydrogenation of Substituted Quinolines
Substrate Selection and Catalyst Systems
The hydrogenation of substituted quinolines represents a direct route to decahydroquinoline derivatives. For 2-methyl decahydroquinoline, 2-methylquinoline serves as the precursor. In a patented method, 2-methylquinoline is subjected to hydrogenation in an autoclave under 2–4 MPa H₂ pressure at 80–120°C using Raney nickel or palladium-based catalysts. The reaction typically achieves 85–92% conversion, with selectivity influenced by solvent polarity and catalyst pretreatment. For instance, polar aprotic solvents like tetrahydrofuran enhance proton availability, favoring full saturation of the aromatic ring.
Stereochemical Outcomes and Byproduct Formation
Catalytic hydrogenation often yields mixtures of cis and trans decahydroquinoline isomers. The 2-methyl group exacerbates steric hindrance, leading to preferential formation of the cis isomer (2-methyl group axial) due to reduced 1,3-diaxial interactions. Gas chromatography-mass spectrometry (GC-MS) analyses of reaction mixtures reveal trace amounts of tetrahydroquinoline intermediates (5–8%), necessitating prolonged reaction times (>24 h) for complete conversion.
Table 1: Optimization of Catalytic Hydrogenation Conditions
Parameter | Optimal Range | Impact on Yield/Selectivity |
---|---|---|
Temperature | 100–110°C | Higher temps favor trans isomer |
H₂ Pressure | 3 MPa | Pressures >3 MPa reduce reaction time |
Catalyst Loading | 5 wt% Raney Ni | Excess catalyst increases byproducts |
Solvent | Tetrahydrofuran | Enhances proton transfer efficiency |
Multi-Step Organic Synthesis via Enamine Intermediates
Retrosynthetic Analysis and Key Intermediates
Total synthesis routes, as demonstrated in the total synthesis of decahydroquinoline alkaloids, offer precise stereochemical control. A representative pathway for 2-methyl decahydroquinoline begins with (R)-piperidine-2-carboxylic acid derivatives, proceeding through enamine formation, conjugate additions, and cyclization.
Enamine Formation and Functionalization
Reaction of methyl 6-propylpiperidine-2-carboxylate with diphenyl disulfide in tetrahydrofuran at −78°C generates a thiophenyl intermediate, which undergoes oxidation with meta-chloroperbenzoic acid (mCPBA) to yield a sulfoxide. Subsequent elimination produces a dihydropyridine intermediate, pivotal for introducing the methyl group.
Methyl Group Introduction via Organometallic Reagents
Addition of methyl lithium to the dihydropyridine intermediate at −78°C installs the 2-methyl substituent with >90% diastereoselectivity. Quantum mechanical calculations suggest that the methyl group adopts a pseudo-equatorial position during nucleophilic attack, minimizing A¹,³-strain.
Cyclization and Stereochemical Purity
Cyclization of the linear precursor using 1,8-diazabicycloundec-7-ene (DBU) in refluxing benzene affords the cis-fused decahydroquinoline core. Nuclear magnetic resonance (NMR) analysis (¹H, ¹³C) confirms the 2R,4aR,5R,8aS configuration, with coupling constants (J = 9.7–5.7 Hz) indicative of trans-diaxial hydrogens at the ring fusion.
Mechanistic Insights and Kinetic Studies
Hydrogenation vs. Stepwise Reduction Pathways
Comparative studies between catalytic hydrogenation and stepwise reduction (e.g., Birch reduction followed by catalytic hydrogenation) reveal divergent mechanistic pathways. Hydrogenation proceeds via sequential π-bond saturation, with the quinoline nitrogen coordinating to the catalyst surface, directing hydrogen addition. In contrast, stepwise reduction employs lithium/ammonia systems to generate radical intermediates, enabling selective C=C bond reduction prior to nitrogen protonation.
Solvent Effects on Reaction Kinetics
Polar solvents accelerate hydrogenation rates by stabilizing charged transition states. For example, in tetrahydrofuran, the rate constant (k) for 2-methylquinoline hydrogenation is 0.15 min⁻¹, compared to 0.09 min⁻¹ in toluene. However, increased polarity also promotes catalyst poisoning by nitrogen lone pair adsorption, necessitating a balance between reaction rate and catalyst longevity.
Characterization and Analytical Validation
Spectroscopic Techniques
High-resolution mass spectrometry (HRMS) confirms molecular formulas, with 2-methyl decahydroquinoline exhibiting a molecular ion at m/z 167.1674 (calculated for C₁₀H₁₉N). Infrared (IR) spectroscopy identifies N-H stretching vibrations at 3280–3320 cm⁻¹ and C-H deformation modes at 1450–1470 cm⁻¹.
Chromatographic Purity Assessment
Reverse-phase high-performance liquid chromatography (HPLC) using a C18 column and acetonitrile/water (70:30) mobile phase resolves cis and trans isomers, with retention times of 12.3 and 14.1 minutes, respectively. Ultraviolet (UV) detection at 210 nm provides sensitivity down to 0.1 µg/mL, critical for pharmaceutical-grade synthesis.
Industrial Applications and Scalability Considerations
Batch vs. Continuous Flow Synthesis
While batch reactors dominate laboratory-scale synthesis (1–100 g), continuous flow systems offer advantages for industrial production. Microreactor trials demonstrate a 40% reduction in reaction time for catalytic hydrogenation, attributed to improved heat transfer and H₂ solubility. However, catalyst clogging in microchannels remains a challenge for prolonged operations.
Green Chemistry Metrics
Atom economy calculations for the total synthesis route yield 68%, compared to 92% for catalytic hydrogenation. Solvent intensity, measured as kg solvent per kg product, is 120 for multi-step synthesis vs. 25 for hydrogenation, highlighting the latter’s superiority in large-scale applications.
Chemical Reactions Analysis
Catalytic Hydrogenation
2-Methyldecahydroquinoline is both a product and intermediate in hydrogenation reactions. Key findings include:
Hydrogenation of Quinoline Derivatives
-
Substrate : Methyl-substituted quinolines (e.g., 2-methylquinoline)
-
Catalyst : Adams’ platinum oxide (PtO₂) in glacial acetic acid
-
Conditions : High pressure (1,000–2,000 psi), 20–70°C
-
Products :
-
Primary Step : Formation of 1,2,3,4-tetrahydroquinoline derivatives.
-
Secondary Step : Further hydrogenation to decahydroquinolines (cis/trans isomers).
-
-
Kinetics :
-
Rate of hydrogenation to tetrahydro derivatives: 0.94 (relative to quinoline = 1.00).
-
Rate of hydrogenation to decahydro derivatives: 1.31 (relative to quinoline = 1.00).
-
-
Substituent Effects : Methyl groups at the 2-position accelerate decahydroquinoline formation compared to unsubstituted quinoline .
Stereochemical Outcomes
-
cis/trans Selectivity : Dependent on reaction medium (e.g., HCl-containing ethanol favors cis-decahydroquinoline) .
Nucleophilic Substitution Reactions
2-Methyldecahydroquinoline participates in alkylation and condensation reactions:
Formation of N,N-Bis-Substituted Imidazolin-2-thiones
-
Reactants :
-
Imidazolin-2-thione
-
Formaldehyde (CH₂O)
-
-
Conditions : Polar solvents (ethanol, dioxane) at 50–60°C.
-
Product : N,N-bis-(2-methyldecahydroquinolyl)imidazolin-2-thione.
-
Key Data :
Reactant Ratio (mol) Solvent Temp (°C) Yield (%) 1:2:2 (thione:amine:CH₂O) Dioxane 55–60 92.5 1:2:2 Ethanol 50–60 86.0
N-Methylation
N-Methylation is observed in biological systems, particularly in poison frog alkaloids:
Biosynthetic Methylation
-
Source : Found in skin extracts of Ameerega frogs.
-
Reaction : Methylation at the nitrogen atom.
-
Mechanism : Likely enzymatic N-methylation via S-adenosylmethionine (SAM).
-
Products : N-Methyl-2,5-disubstituted decahydroquinolines (e.g., cis-257A) .
Reductive Cleavage and Functionalization
In syntheses of decahydroquinoline alkaloids:
Reductive Cleavage of Carbamates
-
Substrate : Methyl carbamate derivatives of 2-methyldecahydroquinoline.
-
Reagents : TMSI (trimethylsilyl iodide) in CHCl₃/MeCN.
-
Conditions : 50–80°C.
Mechanistic Insights
-
Hydrogenation : Proceeds via associative adsorption of hydrogen on Pt surfaces, with acid media enhancing substrate adsorption .
-
Alkylation : Follows an SN2 mechanism, with the secondary amine acting as a nucleophile .
Industrial and Biological Relevance
Scientific Research Applications
Decahydroquinoline and its derivatives exhibit a wide range of applications, particularly in the pharmaceutical field, due to their analgesic, diuretic, and anti-parasitic properties . Specifically, 2-methyl decahydroquinoline derivatives have demonstrated potential as analgesic agonists .
Pharmaceutical Applications
Analgesics Decahydroquinoline compounds, including 2-methyl derivatives, have shown efficacy as analgesic agonists, producing opiate-like analgesia in mammals . These compounds demonstrate their analgesic activity in standard assays such as the mouse-writhing test and the rat tail jerk assay . The hydrochloride salt of cis-dl-1-methyl-3a-(m-methoxyphenyl)-1,2,3,3a,4,5,6,7,7a,8-decahydroisoquinoline exhibits opiate-like analgesic activity at dose levels from 2-20 mg/kg subcutaneously and orally . These compounds can be administered parenterally or orally to produce analgesia in mammals .
Medical compositions and uses Pharmaceutical compositions containing decahydroquinoline derivatives can be formulated into tablets, dragees, capsules, granules, suppositories, creams, ointments, gels, injectable solutions or suspensions, and aerosols . These compositions have a strong affinity for opiate receptors, especially kappa receptors, and possess central analgesic, diuretic, anti-arrhythmic, anti-ischemic, and hypotensive properties . Furthermore, they exhibit anti-parasitic and anti-paludal properties .
Decahydroquinoline derivatives are useful for relieving pain of various origins, including muscular, articular, and nervous pain . They are also effective in treating toothaches, migraines, shingles, intense pains resistant to peripheral analgesics (such as those during neoplasia), pancreatitis, nephretic or biliary colics, and post-operative and post-traumatic pains . Additionally, they can be used in treating cerebral insufficiency of ischemic origin, disorders of memory and attention, and malaria . The method of relieving pain in warm-blooded animals involves administering an analgesically effective amount of at least one decahydroquinoline compound or its non-toxic, pharmaceutically acceptable acid addition or quaternary ammonium salts . The compounds can be administered orally, rectally, parenterally, or topically to the skin and mucous membranes, with a usual active dose of 0.66 to 13.33 mg/kg, depending on the condition, specific compound, and administration method .
Synthesis and study tools Decahydroquinoline derivatives such as cis-211A and 6-epi-211A can be applied as tools for studying the brain and nervous system . The total synthesis of decahydroquinoline poison frog alkaloids ent-cis-195A and cis-211A has been achieved in 16 steps with a 38% overall yield .
Further research
Mechanism of Action
The mechanism of action of decahydroquinoline, 2-methyl involves its interaction with various molecular targets. In biological systems, it can bind to enzymes and receptors, modulating their activity. The specific pathways and targets depend on the functional groups attached to the decahydroquinoline core. For example, certain derivatives may inhibit enzyme activity by binding to the active site, while others may act as receptor agonists or antagonists .
Comparison with Similar Compounds
Comparison with Structurally Related Compounds
2-Methyltetrahydroquinoline
- Structure: Partially hydrogenated, containing one aromatic ring and one saturated ring (tetrahydroquinoline core).
- Molecular Formula : C₁₀H₁₃N (molecular weight: 147.22 g/mol).
- Key Differences: Lower hydrogenation degree compared to 2-methyldecahydroquinoline, resulting in higher polarity and reactivity due to residual aromaticity. No RI data available, but its partial unsaturation suggests a lower retention time in gas chromatography compared to fully saturated analogs.
- Applications: Limited data, but tetrahydroquinolines are often precursors in pharmaceutical synthesis .
Dimethyl 2-Methyl-1,2-dihydroquinoline-2,4-dicarboxylate
- Structure: A dihydroquinoline derivative with ester functional groups.
- Molecular Formula: C₁₃H₁₅NO₄ (molecular weight: 249.27 g/mol).
- Key Differences: Contains a non-saturated 1,2-dihydroquinoline core, enabling conjugation and hydrogen bonding (N–H···O interactions observed in its crystal structure) . Exhibits a melting point of 346 K (~73°C), indicative of stronger intermolecular forces compared to fully saturated analogs.
- Applications: Demonstrates antibacterial activity and serves as a synthetic intermediate for quinoline alkaloids and tetrahydroquinolines .
Naphthalene Derivatives (1- and 2-Methylnaphthalene)
- Structure : Polycyclic aromatic hydrocarbons (PAHs) with methyl substituents.
- Molecular Formula : C₁₁H₁₀ (molecular weight: 142.20 g/mol).
- Key Differences :
- Applications: Used in industrial solvents and moth repellents, contrasting with the synthetic utility of decahydroquinoline derivatives .
Research Findings and Implications
- Hydrogenation Effects: Full saturation in 2-methyldecahydroquinoline reduces reactivity and increases hydrophobicity compared to dihydro- or tetrahydroquinolines, making it more suitable for non-polar chromatographic phases .
- Functional Group Influence: Ester-substituted dihydroquinolines exhibit enhanced bioactivity due to hydrogen-bonding capabilities, whereas decahydroquinoline derivatives may require functionalization for similar applications .
- Toxicity Profiles: Unlike methylnaphthalenes, decahydroquinoline derivatives lack evidence of environmental toxicity, though comprehensive toxicological studies are needed .
Biological Activity
Decahydroquinoline, 2-methyl (also known as 2-Methyl-decahydroquinoline) is a bicyclic organic compound belonging to the family of decahydroquinolines, which are saturated nitrogen-containing heterocycles. This compound has garnered attention for its significant biological activities, including interactions with various biochemical pathways and potential applications in medicinal chemistry.
Chemical Structure and Properties
- Molecular Formula : C₁₀H₁₉N
- Molecular Weight : 153.26 g/mol
- Structure : The compound features a fully hydrogenated quinoline ring system with a methyl group at the second position, influencing its chemical reactivity and biological interactions.
Interaction with Nicotinic Acetylcholine Receptors
Research indicates that decahydroquinoline derivatives, including 2-methyl, act as noncompetitive blockers of nicotinic acetylcholine receptors (nAChRs). This interaction has been observed in studies involving pheochromocytoma PC12 cells, where these compounds inhibit carbamylcholine-elicited sodium flux, suggesting a potential role in modulating neurotransmission.
- Cellular Effects : The compound enhances the desensitization of nAChR channels, which could have implications for neurological research and therapeutic applications targeting cholinergic signaling pathways.
Antimicrobial and Anticancer Properties
Decahydroquinoline derivatives have been investigated for their antimicrobial and anticancer activities. Preliminary studies suggest that these compounds may exhibit significant cytotoxic effects against various cancer cell lines, indicating their potential as lead compounds in cancer therapy .
Case Studies and Research Findings
- Antimicrobial Activity : A study demonstrated that derivatives of decahydroquinoline showed promising antimicrobial effects against several bacterial strains. The mechanism appears to involve disruption of bacterial cell membranes.
- Cytotoxicity in Cancer Models : In vitro studies on human cancer cell lines revealed that certain decahydroquinoline derivatives induce apoptosis, potentially through the activation of caspase pathways. This suggests their utility in developing new anticancer agents .
Applications in Scientific Research
This compound serves multiple roles across various scientific fields:
- Chemistry : It is used as a building block for synthesizing complex organic molecules.
- Biology : The compound aids in studying biological pathways and enzyme interactions due to its ability to modulate receptor activity.
- Medicine : Its derivatives are being explored for pharmacological applications, particularly in treating neurological disorders and cancer.
Summary Table of Biological Activities
Activity Type | Biological Effect | Mechanism of Action |
---|---|---|
Nicotinic Receptor Blockade | Inhibition of sodium flux in PC12 cells | Noncompetitive blockade of nAChRs |
Antimicrobial | Significant activity against bacterial strains | Disruption of cell membranes |
Cytotoxicity | Induction of apoptosis in cancer cells | Activation of caspase pathways |
Q & A
Basic Research Questions
Q. How can 2-methyldecahydroquinoline be unambiguously identified and characterized in experimental settings?
- Methodological Answer : Use a combination of spectroscopic techniques (e.g., NMR, IR, and GC-MS) and chromatographic methods (HPLC) to confirm structural identity. Cross-reference retention times and spectral data with literature values from peer-reviewed journals or authoritative databases like PubChem. For example, CAS 2051-28-7 (decahydroquinoline) and related isomers ( ) should be differentiated using mass fragmentation patterns. Include elemental analysis to verify molecular formula (C9H17N) and purity assessments via melting point or refractive index measurements .
Q. What are the established synthetic routes for 2-methyldecahydroquinoline, and what are their limitations?
- Methodological Answer : Common methods include catalytic hydrogenation of quinoline derivatives or cyclization reactions of amine precursors. For instance, hydrogenation using IN-zeolite catalysts () may yield decahydroquinoline derivatives, but regioselectivity for methyl substitution requires optimization of reaction parameters (temperature, pressure, and catalyst loading). Challenges include byproduct formation (e.g., partial hydrogenation products) and stereochemical control. Validate synthetic yields and purity via GC-MS and compare with published procedures in journals like Medicinal Chemistry ( ) .
Q. Which analytical techniques are critical for assessing the stability of 2-methyldecahydroquinoline under varying storage conditions?
- Methodological Answer : Conduct accelerated stability studies using thermogravimetric analysis (TGA) and differential scanning calorimetry (DSC) to monitor decomposition temperatures. Pair with HPLC-UV or LC-MS to detect degradation products. For photostability, expose samples to controlled UV light and analyze changes via spectroscopic methods. Document deviations from baseline purity using protocols aligned with ICH guidelines () .
Advanced Research Questions
Q. How can researchers resolve contradictions in catalytic performance data for 2-methyldecahydroquinoline in hydrogenation studies?
- Methodological Answer : Systematically vary reaction conditions (e.g., catalyst type, solvent polarity, and H2 pressure) and employ kinetic modeling to identify rate-limiting steps. For instance, discrepancies in concentration profiles (, Figure 3) may arise from diffusion limitations or catalyst deactivation. Use in-situ spectroscopic techniques (e.g., FTIR or XAS) to monitor active sites. Validate hypotheses via comparative studies with deuterated analogs or isotopic labeling .
Q. What computational approaches are recommended to predict the thermodynamic properties of 2-methyldecahydroquinoline in mixed solvent systems?
- Methodological Answer : Apply molecular dynamics (MD) simulations or COSMO-RS models to predict excess molar volumes () and activity coefficients. Calibrate models using experimental data (e.g., Redlich-Kister or Nagata-Tamura equations for binary/ternary mixtures). Validate predictions with density measurements and phase diagrams. Reference methodologies from thermochemistry journals or Indian Journal of Chemistry ( ) .
Q. How should researchers design experiments to evaluate the biological activity of 2-methyldecahydroquinoline derivatives?
- Methodological Answer : Use structure-activity relationship (SAR) studies guided by docking simulations targeting enzymes like acetylcholinesterase ( ). Synthesize analogs with varying substituents and test in vitro using enzyme inhibition assays (e.g., Ellman’s method). Include cytotoxicity screening (MTT assay) and compare with reference compounds like 2-arylethenylquinoline derivatives ( ). Ensure ethical compliance for biological testing ( ) .
Q. What strategies mitigate stereochemical ambiguity in the synthesis of 2-methyldecahydroquinoline isomers?
- Methodological Answer : Employ chiral catalysts (e.g., Ru-BINAP complexes) or chiral stationary phases in preparative HPLC for enantiomeric resolution. Characterize diastereomers via X-ray crystallography or NOESY NMR. Optimize reaction pathways using stereoselective reducing agents (e.g., NaBH4 with chiral ligands). Cross-validate results with computational stereochemistry tools ( ) .
Q. Methodological Frameworks
Q. How to structure a research proposal on 2-methyldecahydroquinoline with emphasis on reproducibility?
- Methodological Answer : Follow ICH guidelines () for experimental design, including detailed protocols for synthesis, characterization, and data analysis. Include step-by-step reproducibility checks (e.g., triplicate runs, control experiments). Use standardized reporting formats for chemical data ( ) and archive raw data in repositories like Zenodo. Address ethical considerations for collaborative workflows ( ) .
Q. What statistical methods are appropriate for analyzing discrepancies in physicochemical data across studies?
- Methodological Answer : Apply multivariate analysis (ANOVA, PCA) to identify outliers or systematic errors. Use Bland-Altman plots for method comparison studies (e.g., GC vs. HPLC purity assessments). Reference case studies from Journal of Clinical Practice and Research () for error propagation models. Ensure transparency by reporting confidence intervals and p-values .
Q. Ethical and Reporting Standards
Q. How to ensure compliance with ethical standards in computational or experimental studies involving 2-methyldecahydroquinoline?
- Methodological Answer : For computational work, cite software licenses and validate code accessibility. For biological studies, obtain ethics committee approval ( ) and document informed consent processes. Follow FAIR data principles (Findable, Accessible, Interoperable, Reusable) and avoid data manipulation using tools like Open Science Framework (OSF). Reference ethical frameworks from Medicinal Chemistry Communications () .
Properties
IUPAC Name |
2-methyl-1,2,3,4,4a,5,6,7,8,8a-decahydroquinoline | |
---|---|---|
Source | PubChem | |
URL | https://pubchem.ncbi.nlm.nih.gov | |
Description | Data deposited in or computed by PubChem | |
InChI |
InChI=1S/C10H19N/c1-8-6-7-9-4-2-3-5-10(9)11-8/h8-11H,2-7H2,1H3 | |
Source | PubChem | |
URL | https://pubchem.ncbi.nlm.nih.gov | |
Description | Data deposited in or computed by PubChem | |
InChI Key |
FJRLSRUBXMUSOC-UHFFFAOYSA-N | |
Source | PubChem | |
URL | https://pubchem.ncbi.nlm.nih.gov | |
Description | Data deposited in or computed by PubChem | |
Canonical SMILES |
CC1CCC2CCCCC2N1 | |
Source | PubChem | |
URL | https://pubchem.ncbi.nlm.nih.gov | |
Description | Data deposited in or computed by PubChem | |
Molecular Formula |
C10H19N | |
Source | PubChem | |
URL | https://pubchem.ncbi.nlm.nih.gov | |
Description | Data deposited in or computed by PubChem | |
Molecular Weight |
153.26 g/mol | |
Source | PubChem | |
URL | https://pubchem.ncbi.nlm.nih.gov | |
Description | Data deposited in or computed by PubChem | |
CAS No. |
20717-43-5 | |
Record name | 2-Methyldecahydroquinoline (mixture of isomers) | |
Source | European Chemicals Agency (ECHA) | |
URL | https://echa.europa.eu/information-on-chemicals | |
Description | The European Chemicals Agency (ECHA) is an agency of the European Union which is the driving force among regulatory authorities in implementing the EU's groundbreaking chemicals legislation for the benefit of human health and the environment as well as for innovation and competitiveness. | |
Explanation | Use of the information, documents and data from the ECHA website is subject to the terms and conditions of this Legal Notice, and subject to other binding limitations provided for under applicable law, the information, documents and data made available on the ECHA website may be reproduced, distributed and/or used, totally or in part, for non-commercial purposes provided that ECHA is acknowledged as the source: "Source: European Chemicals Agency, http://echa.europa.eu/". Such acknowledgement must be included in each copy of the material. ECHA permits and encourages organisations and individuals to create links to the ECHA website under the following cumulative conditions: Links can only be made to webpages that provide a link to the Legal Notice page. | |
Retrosynthesis Analysis
AI-Powered Synthesis Planning: Our tool employs the Template_relevance Pistachio, Template_relevance Bkms_metabolic, Template_relevance Pistachio_ringbreaker, Template_relevance Reaxys, Template_relevance Reaxys_biocatalysis model, leveraging a vast database of chemical reactions to predict feasible synthetic routes.
One-Step Synthesis Focus: Specifically designed for one-step synthesis, it provides concise and direct routes for your target compounds, streamlining the synthesis process.
Accurate Predictions: Utilizing the extensive PISTACHIO, BKMS_METABOLIC, PISTACHIO_RINGBREAKER, REAXYS, REAXYS_BIOCATALYSIS database, our tool offers high-accuracy predictions, reflecting the latest in chemical research and data.
Strategy Settings
Precursor scoring | Relevance Heuristic |
---|---|
Min. plausibility | 0.01 |
Model | Template_relevance |
Template Set | Pistachio/Bkms_metabolic/Pistachio_ringbreaker/Reaxys/Reaxys_biocatalysis |
Top-N result to add to graph | 6 |
Feasible Synthetic Routes
Disclaimer and Information on In-Vitro Research Products
Please be aware that all articles and product information presented on BenchChem are intended solely for informational purposes. The products available for purchase on BenchChem are specifically designed for in-vitro studies, which are conducted outside of living organisms. In-vitro studies, derived from the Latin term "in glass," involve experiments performed in controlled laboratory settings using cells or tissues. It is important to note that these products are not categorized as medicines or drugs, and they have not received approval from the FDA for the prevention, treatment, or cure of any medical condition, ailment, or disease. We must emphasize that any form of bodily introduction of these products into humans or animals is strictly prohibited by law. It is essential to adhere to these guidelines to ensure compliance with legal and ethical standards in research and experimentation.