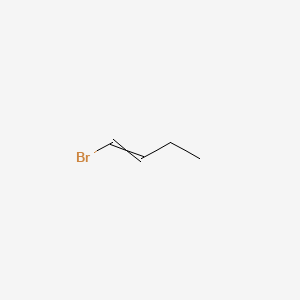
1-Bromobut-1-ene
- Click on QUICK INQUIRY to receive a quote from our team of experts.
- With the quality product at a COMPETITIVE price, you can focus more on your research.
Overview
Description
1-Bromobut-1-ene, also known as 4-bromo-1-butene, is an organobromine compound with the molecular formula C4H7Br. It is a colorless liquid that is used in various chemical synthesis processes. This compound is particularly notable for its reactivity, making it a valuable intermediate in organic synthesis.
Preparation Methods
Synthetic Routes and Reaction Conditions
1-Bromobut-1-ene can be synthesized through several methods. One common method involves the addition of hydrogen bromide to butadiene. This reaction typically requires a catalyst and is carried out under controlled temperature and pressure conditions to ensure the desired product is obtained.
Another method involves the bromination of butene using bromine in the presence of a solvent such as carbon tetrachloride. This reaction is usually performed at room temperature and yields bromobutene as the primary product.
Industrial Production Methods
In industrial settings, bromobutene is often produced through the bromination of butene. This process involves the use of large-scale reactors and precise control of reaction conditions to maximize yield and purity. The product is then purified through distillation and other separation techniques to remove any impurities.
Chemical Reactions Analysis
Types of Reactions
1-Bromobut-1-ene undergoes various types of chemical reactions, including:
Substitution Reactions: this compound can participate in nucleophilic substitution reactions where the bromine atom is replaced by another nucleophile.
Elimination Reactions: Under basic conditions, bromobutene can undergo elimination reactions to form butadiene.
Addition Reactions: this compound can react with halogens or hydrogen halides to form dihalogenated or halogenated products.
Common Reagents and Conditions
Nucleophilic Substitution: Common reagents include sodium hydroxide or potassium hydroxide, typically in an aqueous or alcoholic solution.
Elimination: Strong bases such as sodium ethoxide or potassium tert-butoxide are used.
Addition: Halogens like chlorine or bromine, and hydrogen halides like hydrogen chloride or hydrogen bromide, are used under controlled conditions.
Major Products
Substitution: Products include various substituted butenes depending on the nucleophile used.
Elimination: The major product is butadiene.
Addition: Products include dihalogenated butanes or halogenated butenes.
Scientific Research Applications
1-Bromobut-1-ene has several applications in scientific research:
Chemistry: It is used as an intermediate in the synthesis of various organic compounds, including pharmaceuticals and agrochemicals.
Biology: this compound derivatives are studied for their potential biological activities, including antimicrobial and anticancer properties.
Medicine: It is used in the synthesis of active pharmaceutical ingredients and as a building block for drug development.
Industry: this compound is used in the production of polymers, resins, and other industrial chemicals.
Mechanism of Action
The mechanism of action of bromobutene involves its reactivity as an electrophile due to the presence of the bromine atom. In nucleophilic substitution reactions, the bromine atom is replaced by a nucleophile, resulting in the formation of a new compound. In elimination reactions, the bromine atom is removed along with a hydrogen atom, leading to the formation of a double bond and the production of butadiene.
Comparison with Similar Compounds
Similar Compounds
1-Bromobutane: Similar in structure but with the bromine atom at a different position.
2-Bromobutane: Another isomer with the bromine atom on the second carbon.
4-Chloro-1-butene: Similar structure but with a chlorine atom instead of bromine.
Uniqueness
1-Bromobut-1-ene is unique due to its specific reactivity and position of the bromine atom, which allows for selective reactions and the formation of specific products. Its ability to undergo both substitution and elimination reactions makes it a versatile compound in organic synthesis.
Properties
Molecular Formula |
C4H7B |
---|---|
Molecular Weight |
135 g/mol |
IUPAC Name |
1-bromobut-1-ene |
InChI |
InChI=1S/C4H7Br/c1-2-3-4-5/h3-4H,2H2,1H3 |
InChI Key |
IUXHPSPHPKXTPA-UHFFFAOYSA-N |
SMILES |
CCC=CBr |
Canonical SMILES |
CCC=CBr |
Origin of Product |
United States |
Disclaimer and Information on In-Vitro Research Products
Please be aware that all articles and product information presented on BenchChem are intended solely for informational purposes. The products available for purchase on BenchChem are specifically designed for in-vitro studies, which are conducted outside of living organisms. In-vitro studies, derived from the Latin term "in glass," involve experiments performed in controlled laboratory settings using cells or tissues. It is important to note that these products are not categorized as medicines or drugs, and they have not received approval from the FDA for the prevention, treatment, or cure of any medical condition, ailment, or disease. We must emphasize that any form of bodily introduction of these products into humans or animals is strictly prohibited by law. It is essential to adhere to these guidelines to ensure compliance with legal and ethical standards in research and experimentation.