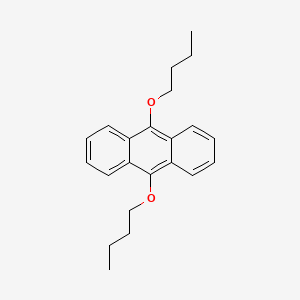
9,10-Dibutoxyanthracene
Overview
Description
9,10-Dibutoxyanthracene (C₂₂H₂₆O₂, molecular weight: 322.44 g/mol) is a disubstituted anthracene derivative with butoxy groups at the 9, and 10 positions. It is a yellow crystalline powder with a melting point of 105–125°C and serves as a photosensitizer in UV cationic polymerization due to its unique absorption in long UV wavelengths (>400 nm) . Its photodegradation mechanism involves the formation of an endoperoxide species upon reaction with atmospheric oxygen, enabling its application as a photo-induced oxygen scavenger . The compound enhances polymerization efficiency, improves surface properties of cured products, and complies with regulatory standards in industrial applications .
Preparation Methods
Synthetic Routes and Reaction Conditions: The synthesis of 9,10-Dibutoxyanthracene typically involves the reaction of anthraquinone with n-butyryl chloride in the presence of sodium hydroxide and sodium hydrosulfite. This reaction yields an intermediate, which is then reduced to form this compound .
Industrial Production Methods: While specific industrial production methods for this compound are not extensively documented, the general approach involves large-scale reactions similar to the laboratory synthesis, with optimizations for yield and purity.
Types of Reactions:
Photodegradation: The compound is known to degrade upon exposure to light, especially in the presence of air, resulting in secondary decomposition products.
Common Reagents and Conditions:
Oxidation: Singlet oxygen is a common reagent used in the oxidation of this compound.
Photodegradation: Light exposure in the presence of air facilitates the degradation process.
Major Products Formed:
Endoperoxides: Formed during the oxidation of this compound.
Secondary Decomposition Products: Result from the photodegradation of the endoperoxides.
Scientific Research Applications
Photopolymerization
DBA is widely recognized for its application as an electron transfer sensitizer in photopolymerization processes. Its favorable optical properties allow it to effectively absorb light in the ultraviolet (UV) region, particularly between 360-400 nm, which is crucial for initiating polymerization reactions when exposed to UV light sources such as LEDs .
Photoinitiators in Coatings and Inks
Due to its photochemical properties, DBA is employed as a photoinitiator in UV-curable inks and coatings. The compound's ability to generate free radicals upon light exposure makes it suitable for applications in graphic arts and protective coatings where rapid curing is essential .
Advantages
- Fast curing times enhance production efficiency.
- High absorption in the UVA range allows for effective use with various light sources.
Organic Light Emitting Diodes (OLEDs)
DBA's optical characteristics make it a candidate for use in OLEDs . Its ability to participate in triplet-triplet annihilation processes enhances the efficiency of light emission in OLED devices. The incorporation of DBA into OLED materials can improve their performance by increasing the quantum yield of emitted light .
Advanced Material Development
Research indicates that DBA can be utilized in developing advanced materials such as:
- Photoresists : Used for fine pattern formation in semiconductor manufacturing.
- UVA Absorbers : Protects against UV-induced degradation, making it valuable in cosmetic formulations aimed at preventing skin aging .
Photodegradation Studies
Understanding the photodegradation of DBA is crucial for optimizing its applications. Studies have shown that prolonged exposure to light can lead to significant decomposition, impacting its effectiveness as a sensitizer. Research has identified specific degradation pathways involving the formation of endoperoxide intermediates and their subsequent breakdown products .
Application Area | Key Features | Potential Benefits |
---|---|---|
Photopolymerization | Electron transfer sensitizer | Rapid curing, high efficiency |
Coatings and Inks | Photoinitiator | Improved durability and performance |
OLEDs | Enhances light emission | Increased quantum yield |
Advanced Materials | Photoresists and UVA absorbers | Versatile applications |
Case Studies
- Photopolymerization Efficiency : A study demonstrated that DBA significantly enhances the polymerization rate when used with specific monomers under UV light, showcasing its effectiveness as a photoinitiator .
- Photodegradation Pathways : Research outlined the mechanisms through which DBA degrades upon UV exposure, identifying key intermediates that could be targeted to improve stability during industrial applications .
- OLED Performance : Experimental results indicated that incorporating DBA into OLED formulations led to improved color purity and brightness, validating its role as a beneficial additive in electronic materials .
Mechanism of Action
The primary mechanism by which 9,10-Dibutoxyanthracene exerts its effects involves the absorption of light, leading to the generation of singlet oxygen. This reactive oxygen species can then participate in various chemical reactions, including the formation of endoperoxides. The molecular targets and pathways involved in these processes are primarily related to the compound’s ability to transfer energy and electrons .
Comparison with Similar Compounds
Structural and Functional Differences
Substituent Effects on Properties
The photophysical and chemical behaviors of 9,10-disubstituted anthracenes are highly dependent on the electronic nature and steric bulk of substituents:
- Electron-donating groups (e.g., butoxy) : Enhance photosensitizing capabilities by lowering singlet-triplet energy gaps, as seen in 9,10-Dibutoxyanthracene.
- Electron-withdrawing groups (e.g., bromo) : Increase electrophilicity, making compounds like 9,10-Dibromoanthracene (C₁₄H₈Br₂, 336.02 g/mol) reactive intermediates in Suzuki couplings .
- Heteroaromatic rings (e.g., thiophene, furan) : Influence aggregation-induced emission (AIE) properties. For example, 9,10-di(thiophen-2-yl)anthracene (DTA) exhibits AIE with a fluorescence quantum yield (Φ) of 0.009 in DMF, while 9,10-di(furan-2-yl)anthracene (DFA) shows a Φ of 0.010 .
Aggregation-Induced Emission (AIE) vs. Aggregation-Caused Quenching (ACQ)
- AIE Compounds (DTA, DFA, DPA) : Exhibit weak emission in dilute solutions but strong fluorescence in aggregated states (e.g., Φ increases 19-fold for DFA at 90% water content in DMF/H₂O) .
- ACQ Compound (DBPA) : Shows strong fluorescence in solution due to reduced steric hindrance from Boc groups, but quenching in aggregates .
Photochemical Stability and Degradation
- This compound : Undergoes photodegradation via endoperoxide formation, enabling oxygen scavenging .
- 9,10-Dibromoanthracene : Stable under UV light but serves as a precursor for cross-coupling reactions .
- AIEgens (DTA, DFA) : Resistant to photobleaching, making them suitable for long-term bioimaging .
Key Research Findings
This compound outperforms other photosensitizers in thick-film polymerization due to its broad UV absorption and energy-transfer efficiency .
DBPA ’s ACQ behavior is attributed to planarization of the anthracene core in solution, while steric hindrance in aggregates disrupts emission .
DFA exhibits a 40 nm red shift in polar solvents (e.g., DMF) due to J-aggregation facilitated by hydrogen bonding .
Biological Activity
9,10-Dibutoxyanthracene (DBA) is a derivative of anthracene, characterized by the presence of butoxy groups at the 9 and 10 positions of the anthracene core. This compound has garnered attention for its biological activity , particularly in photodynamic therapy and as an electron transfer sensitizer in photopolymerization processes. Its unique optical properties and reactivity with oxygen make it a subject of interest in various scientific fields.
This compound primarily interacts with oxygen to form an endoperoxide species . This reaction is facilitated by light exposure, which promotes the formation of singlet oxygen, a reactive species that can induce cellular damage or therapeutic effects in biological systems .
Photoreactivity
The compound absorbs light effectively in the range of 360–400 nm, making it suitable for applications as a UVA absorber . This absorption range aligns with wavelengths that contribute to skin aging, thus positioning DBA as a potential agent in photoprotection strategies .
Decomposition and Secondary Products
Upon prolonged irradiation, DBA can decompose into secondary products, including various decomposition products identified through spectroscopic techniques. The mechanism of photodegradation involves the formation of endoperoxides that subsequently break down under light exposure .
Applications in Photodynamic Therapy
DBA's ability to generate singlet oxygen upon excitation makes it a promising candidate for photodynamic therapy (PDT) . In PDT, singlet oxygen can selectively induce cytotoxic effects in target cells, such as cancer cells, while minimizing damage to surrounding healthy tissue .
Research Findings
Research has demonstrated that DBA can act as a photo-induced oxygen scavenger , which may enhance its efficacy in PDT by modulating local oxygen levels during treatment. Studies have shown that DBA's reactivity with singlet oxygen is influenced by its substituents, affecting its overall biological activity .
Case Studies
- Photodegradation Studies : Research conducted on DBA's photodegradation highlighted its stability under certain conditions and the formation of identifiable secondary products. These findings are crucial for understanding its long-term behavior in biological systems and potential therapeutic applications .
- Therapeutic Applications : In vitro studies have indicated that DBA can effectively induce cell death in specific cancer cell lines when activated by light, showcasing its potential role in targeted cancer therapies .
Comparative Analysis
The table below compares this compound with other anthracene derivatives regarding their biological activity and applications:
Compound | Absorption Range (nm) | Biological Activity | Application Area |
---|---|---|---|
This compound | 360-400 | Singlet oxygen generation | Photodynamic therapy |
9,10-Diphenylanthracene | 350-400 | High fluorescence | Triplet–triplet annihilation |
9,10-Dimethylanthracene | 340-420 | Moderate singlet oxygen generation | Photopolymerization |
Q & A
Q. What experimental methods are recommended for synthesizing 9,10-Dibutoxyanthracene, and how can purity be optimized?
Basic Research Question
this compound is synthesized via nucleophilic substitution, where anthraquinone derivatives are alkoxylated using butanol under acidic or basic conditions. Key steps include:
- Reagent Preparation : Use anhydrous conditions to avoid hydrolysis of alkoxy groups.
- Purification : Recrystallization from ethanol or column chromatography (silica gel, hexane/ethyl acetate eluent) ensures high purity (>98%) .
- Characterization : Confirm structure via H NMR (δ 1.0–1.6 ppm for butyl CH/CH, δ 6.8–8.5 ppm for aromatic protons) and FT-IR (C-O-C stretch at ~1250 cm) .
Q. How can researchers characterize the photodegradation pathways of this compound in aerobic conditions?
Advanced Research Question
Photodegradation studies require controlled oxygen exposure and UV irradiation (e.g., 365 nm LED). Methodological steps include:
- Experimental Setup : Use quartz cuvettes under inert atmosphere with controlled O introduction. Monitor reaction via UV-Vis spectroscopy (absorption decay at λ ~400 nm) .
- Product Isolation : Secondary decomposition products (e.g., anthraquinone derivatives) are isolated via HPLC (C18 column, acetonitrile/water gradient) and identified via GC-MS .
- Mechanistic Validation : Singlet oxygen (O) scavengers (e.g., β-carotene) and computational modeling (TD-DFT for singlet-triplet energy gaps) validate the proposed endoperoxide intermediate .
Table 1 : Key Photodegradation Parameters
Parameter | Value/Condition | Reference |
---|---|---|
UV Wavelength | 365 nm | |
Reaction Half-Life (O) | ~45 min (1 atm, 25°C) | |
Major Byproduct | 9,10-Anthraquinone derivative |
Q. What role does this compound play in UV-cationic polymerization, and how is its efficiency quantified?
Basic Research Question
As a photoinitiator, this compound generates cationic species under UV light. Key considerations:
- Reaction Setup : Combine with iodonium salts (e.g., diphenyl iodonium hexafluorophosphate) in epoxy resins. Irradiate at 350–400 nm .
- Efficiency Metrics : Measure polymerization rate via real-time FT-IR (disappearance of epoxy C-O stretch at ~915 cm) or photo-DSC (exothermic peak analysis) .
- Optimization : Adjust initiator concentration (0.5–2 wt%) and light intensity (10–50 mW/cm) to balance cure speed and final polymer stability .
Q. How do computational studies support the experimental analysis of this compound’s photophysical properties?
Advanced Research Question
TD-DFT calculations clarify electronic transitions and oxygen-scavenging mechanisms:
- Singlet-Triplet Gaps : Calculate energy differences between S and T states (~0.8 eV) to predict O quenching efficiency .
- Molecular Orbital Analysis : HOMO-LUMO gaps (~3.2 eV) correlate with UV-Vis absorption maxima (λ ~380 nm) .
- Reaction Pathway Modeling : Simulate endoperoxide formation kinetics using Eyring equation parameters (Δ‡H and Δ‡S) .
Q. What strategies mitigate instability issues in this compound during long-term storage?
Advanced Research Question
Degradation is minimized via:
- Storage Conditions : Seal in amber vials under argon (-20°C), with desiccants to prevent moisture absorption .
- Stability Monitoring : Track purity via periodic HPLC analysis (retention time shift indicates degradation) .
- Additives : Stabilize with radical inhibitors (e.g., BHT at 0.1 wt%) in polymer formulations .
Q. How does solvent polarity affect the reactivity of this compound in oxygen-scavenging applications?
Advanced Research Question
Solvent choice impacts reaction kinetics and byproduct profiles:
- Polar Protic Solvents (e.g., MeOH) : Accelerate endoperoxide formation but promote hydrolysis side reactions.
- Nonpolar Solvents (e.g., Toluene) : Slow degradation rates but improve selectivity for anthraquinone derivatives .
- Quantitative Analysis : Use stopped-flow spectroscopy to measure O quenching rates (k ~1.5 × 10 Ms in DCM) .
Q. What analytical techniques resolve contradictions in reported photodegradation byproducts?
Advanced Research Question
Discrepancies arise from varying O concentrations or light sources. Resolution methods include:
- High-Resolution Mass Spectrometry (HRMS) : Confirm molecular formulas of minor byproducts (e.g., m/z 298.12 for dihydroxyanthracene) .
- Isotopic Labeling : Use O to trace oxygen incorporation in endoperoxide intermediates .
- Cross-Study Comparison : Normalize data to photon flux (measured by actinometry) for reproducibility .
Properties
IUPAC Name |
9,10-dibutoxyanthracene | |
---|---|---|
Source | PubChem | |
URL | https://pubchem.ncbi.nlm.nih.gov | |
Description | Data deposited in or computed by PubChem | |
InChI |
InChI=1S/C22H26O2/c1-3-5-15-23-21-17-11-7-9-13-19(17)22(24-16-6-4-2)20-14-10-8-12-18(20)21/h7-14H,3-6,15-16H2,1-2H3 | |
Source | PubChem | |
URL | https://pubchem.ncbi.nlm.nih.gov | |
Description | Data deposited in or computed by PubChem | |
InChI Key |
KSMGAOMUPSQGTB-UHFFFAOYSA-N | |
Source | PubChem | |
URL | https://pubchem.ncbi.nlm.nih.gov | |
Description | Data deposited in or computed by PubChem | |
Canonical SMILES |
CCCCOC1=C2C=CC=CC2=C(C3=CC=CC=C31)OCCCC | |
Source | PubChem | |
URL | https://pubchem.ncbi.nlm.nih.gov | |
Description | Data deposited in or computed by PubChem | |
Molecular Formula |
C22H26O2 | |
Source | PubChem | |
URL | https://pubchem.ncbi.nlm.nih.gov | |
Description | Data deposited in or computed by PubChem | |
DSSTOX Substance ID |
DTXSID20621211 | |
Record name | 9,10-Dibutoxyanthracene | |
Source | EPA DSSTox | |
URL | https://comptox.epa.gov/dashboard/DTXSID20621211 | |
Description | DSSTox provides a high quality public chemistry resource for supporting improved predictive toxicology. | |
Molecular Weight |
322.4 g/mol | |
Source | PubChem | |
URL | https://pubchem.ncbi.nlm.nih.gov | |
Description | Data deposited in or computed by PubChem | |
CAS No. |
76275-14-4 | |
Record name | 9,10-Dibutoxyanthracene | |
Source | EPA DSSTox | |
URL | https://comptox.epa.gov/dashboard/DTXSID20621211 | |
Description | DSSTox provides a high quality public chemistry resource for supporting improved predictive toxicology. | |
Retrosynthesis Analysis
AI-Powered Synthesis Planning: Our tool employs the Template_relevance Pistachio, Template_relevance Bkms_metabolic, Template_relevance Pistachio_ringbreaker, Template_relevance Reaxys, Template_relevance Reaxys_biocatalysis model, leveraging a vast database of chemical reactions to predict feasible synthetic routes.
One-Step Synthesis Focus: Specifically designed for one-step synthesis, it provides concise and direct routes for your target compounds, streamlining the synthesis process.
Accurate Predictions: Utilizing the extensive PISTACHIO, BKMS_METABOLIC, PISTACHIO_RINGBREAKER, REAXYS, REAXYS_BIOCATALYSIS database, our tool offers high-accuracy predictions, reflecting the latest in chemical research and data.
Strategy Settings
Precursor scoring | Relevance Heuristic |
---|---|
Min. plausibility | 0.01 |
Model | Template_relevance |
Template Set | Pistachio/Bkms_metabolic/Pistachio_ringbreaker/Reaxys/Reaxys_biocatalysis |
Top-N result to add to graph | 6 |
Feasible Synthetic Routes
Disclaimer and Information on In-Vitro Research Products
Please be aware that all articles and product information presented on BenchChem are intended solely for informational purposes. The products available for purchase on BenchChem are specifically designed for in-vitro studies, which are conducted outside of living organisms. In-vitro studies, derived from the Latin term "in glass," involve experiments performed in controlled laboratory settings using cells or tissues. It is important to note that these products are not categorized as medicines or drugs, and they have not received approval from the FDA for the prevention, treatment, or cure of any medical condition, ailment, or disease. We must emphasize that any form of bodily introduction of these products into humans or animals is strictly prohibited by law. It is essential to adhere to these guidelines to ensure compliance with legal and ethical standards in research and experimentation.