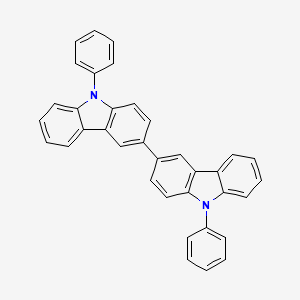
9,9'-Diphenyl-9H,9'H-3,3'-bicarbazole
Overview
Description
9,9'-Diphenyl-9H,9'H-3,3'-bicarbazole is a useful research compound. Its molecular formula is C36H24N2 and its molecular weight is 484.6 g/mol. The purity is usually 95%.
The exact mass of the compound this compound is unknown and the complexity rating of the compound is unknown. The United Nations designated GHS hazard class pictogram is Irritant, and the GHS signal word is WarningThe storage condition is unknown. Please store according to label instructions upon receipt of goods.
BenchChem offers high-quality this compound suitable for many research applications. Different packaging options are available to accommodate customers' requirements. Please inquire for more information about this compound including the price, delivery time, and more detailed information at info@benchchem.com.
Mechanism of Action
Target of Action
The primary target of 9,9’-Diphenyl-9H,9’H-3,3’-bicarbazole is the organic light-emitting diode (OLED) materials . It serves as a source of electrons to create exciplexes .
Mode of Action
9,9’-Diphenyl-9H,9’H-3,3’-bicarbazole interacts with its targets by serving as a source of electrons . This interaction results in the creation of exciplexes, which can function as either an emitting layer or a host material in fluorescent and phosphorescent OLED devices .
Biochemical Pathways
The compound affects the biochemical pathways involved in the operation of OLED devices . The downstream effects include the emission of light in the devices .
Pharmacokinetics
It is soluble in benzene, ethanol, and chloroform, and slightly soluble in ether .
Result of Action
The molecular and cellular effects of 9,9’-Diphenyl-9H,9’H-3,3’-bicarbazole’s action are observed in the performance of OLED devices . Its interaction with the device materials results in the emission of light .
Action Environment
Environmental factors such as temperature and exposure to light can influence the action, efficacy, and stability of 9,9’-Diphenyl-9H,9’H-3,3’-bicarbazole. It has a melting point of 204.0 to 208.0 °C and a boiling point of 721.9±60.0 °C (Predicted) . Proper storage and handling conditions, such as avoiding contact with oxidizing agents, strong acids, and strong bases, are necessary to prevent hazardous situations .
Biochemical Analysis
Biochemical Properties
It is known that this compound can interact with other molecules in the context of OLED devices . The nature of these interactions is primarily electronic, as 9,9’-Diphenyl-9H,9’H-3,3’-bicarbazole can donate electrons to form exciplexes .
Molecular Mechanism
The molecular mechanism of action of 9,9’-Diphenyl-9H,9’H-3,3’-bicarbazole involves its ability to donate electrons and form exciplexes in the context of OLED devices . This process is crucial for the high device performance of these OLEDs .
Temporal Effects in Laboratory Settings
It is known that this compound is used in the manufacture of OLED devices, suggesting that it has good stability under the conditions used in these processes .
Properties
IUPAC Name |
9-phenyl-3-(9-phenylcarbazol-3-yl)carbazole | |
---|---|---|
Source | PubChem | |
URL | https://pubchem.ncbi.nlm.nih.gov | |
Description | Data deposited in or computed by PubChem | |
InChI |
InChI=1S/C36H24N2/c1-3-11-27(12-4-1)37-33-17-9-7-15-29(33)31-23-25(19-21-35(31)37)26-20-22-36-32(24-26)30-16-8-10-18-34(30)38(36)28-13-5-2-6-14-28/h1-24H | |
Source | PubChem | |
URL | https://pubchem.ncbi.nlm.nih.gov | |
Description | Data deposited in or computed by PubChem | |
InChI Key |
MHTPESFJWCJELK-UHFFFAOYSA-N | |
Source | PubChem | |
URL | https://pubchem.ncbi.nlm.nih.gov | |
Description | Data deposited in or computed by PubChem | |
Canonical SMILES |
C1=CC=C(C=C1)N2C3=C(C=C(C=C3)C4=CC5=C(C=C4)N(C6=CC=CC=C65)C7=CC=CC=C7)C8=CC=CC=C82 | |
Source | PubChem | |
URL | https://pubchem.ncbi.nlm.nih.gov | |
Description | Data deposited in or computed by PubChem | |
Molecular Formula |
C36H24N2 | |
Source | PubChem | |
URL | https://pubchem.ncbi.nlm.nih.gov | |
Description | Data deposited in or computed by PubChem | |
DSSTOX Substance ID |
DTXSID901347599 | |
Record name | 9-Phenyl-3-(9-phenylcarbazol-3-yl)carbazole | |
Source | EPA DSSTox | |
URL | https://comptox.epa.gov/dashboard/DTXSID901347599 | |
Description | DSSTox provides a high quality public chemistry resource for supporting improved predictive toxicology. | |
Molecular Weight |
484.6 g/mol | |
Source | PubChem | |
URL | https://pubchem.ncbi.nlm.nih.gov | |
Description | Data deposited in or computed by PubChem | |
CAS No. |
57102-62-2 | |
Record name | 9-Phenyl-3-(9-phenylcarbazol-3-yl)carbazole | |
Source | EPA DSSTox | |
URL | https://comptox.epa.gov/dashboard/DTXSID901347599 | |
Description | DSSTox provides a high quality public chemistry resource for supporting improved predictive toxicology. | |
Record name | 3,3'-Bi-9H-carbazole, 9,9'-diphenyl- | |
Source | European Chemicals Agency (ECHA) | |
URL | https://echa.europa.eu/information-on-chemicals | |
Description | The European Chemicals Agency (ECHA) is an agency of the European Union which is the driving force among regulatory authorities in implementing the EU's groundbreaking chemicals legislation for the benefit of human health and the environment as well as for innovation and competitiveness. | |
Explanation | Use of the information, documents and data from the ECHA website is subject to the terms and conditions of this Legal Notice, and subject to other binding limitations provided for under applicable law, the information, documents and data made available on the ECHA website may be reproduced, distributed and/or used, totally or in part, for non-commercial purposes provided that ECHA is acknowledged as the source: "Source: European Chemicals Agency, http://echa.europa.eu/". Such acknowledgement must be included in each copy of the material. ECHA permits and encourages organisations and individuals to create links to the ECHA website under the following cumulative conditions: Links can only be made to webpages that provide a link to the Legal Notice page. | |
Retrosynthesis Analysis
AI-Powered Synthesis Planning: Our tool employs the Template_relevance Pistachio, Template_relevance Bkms_metabolic, Template_relevance Pistachio_ringbreaker, Template_relevance Reaxys, Template_relevance Reaxys_biocatalysis model, leveraging a vast database of chemical reactions to predict feasible synthetic routes.
One-Step Synthesis Focus: Specifically designed for one-step synthesis, it provides concise and direct routes for your target compounds, streamlining the synthesis process.
Accurate Predictions: Utilizing the extensive PISTACHIO, BKMS_METABOLIC, PISTACHIO_RINGBREAKER, REAXYS, REAXYS_BIOCATALYSIS database, our tool offers high-accuracy predictions, reflecting the latest in chemical research and data.
Strategy Settings
Precursor scoring | Relevance Heuristic |
---|---|
Min. plausibility | 0.01 |
Model | Template_relevance |
Template Set | Pistachio/Bkms_metabolic/Pistachio_ringbreaker/Reaxys/Reaxys_biocatalysis |
Top-N result to add to graph | 6 |
Feasible Synthetic Routes
Disclaimer and Information on In-Vitro Research Products
Please be aware that all articles and product information presented on BenchChem are intended solely for informational purposes. The products available for purchase on BenchChem are specifically designed for in-vitro studies, which are conducted outside of living organisms. In-vitro studies, derived from the Latin term "in glass," involve experiments performed in controlled laboratory settings using cells or tissues. It is important to note that these products are not categorized as medicines or drugs, and they have not received approval from the FDA for the prevention, treatment, or cure of any medical condition, ailment, or disease. We must emphasize that any form of bodily introduction of these products into humans or animals is strictly prohibited by law. It is essential to adhere to these guidelines to ensure compliance with legal and ethical standards in research and experimentation.