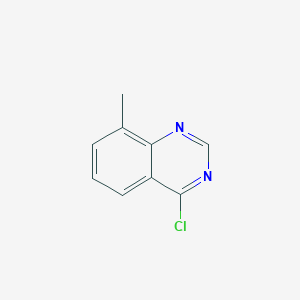
4-Chloro-8-methylquinazoline
Overview
Description
4-Chloro-8-methylquinazoline (CAS 58421-80-0) is a halogenated quinazoline derivative with the molecular formula C₉H₇ClN₂ and a molecular weight of 178.62 g/mol . Its structure features a chlorine atom at position 4 and a methyl group at position 8, as depicted in the SMILES notation Cc1cccc2c1ncnc2Cl . This compound is synthesized via chlorination of 8-methylquinazolin-4(3H)-one using phosphorus oxychloride (POCl₃), yielding 81–82% under optimized conditions . It serves as a key intermediate in Suzuki-Miyaura cross-coupling reactions to generate 4-arylquinazoline derivatives, such as 4-(4-tert-butylphenyl)-8-methylquinazoline, which exhibit antibacterial activity against methicillin-resistant Staphylococcus aureus (MRSA) .
The compound’s moderate lipophilicity (XLogP3 = 2.9) and hydrogen-bond acceptor count (2) suggest balanced solubility and membrane permeability, making it suitable for pharmaceutical applications . Its NMR and LCMS data confirm structural integrity: ¹H NMR (CDCl₃) δ 2.75 (s, 3H, CH₃), 7.50–8.13 (m, aromatic H) and LCMS m/z 179 [M+H]⁺ .
Biological Activity
4-Chloro-8-methylquinazoline is a compound belonging to the quinazoline family, which has garnered attention due to its diverse biological activities, particularly in medicinal chemistry. This article explores its biological activity, including anticancer and antimicrobial properties, mechanisms of action, and relevant research findings.
Chemical Structure and Properties
The molecular formula of this compound is , with a molecular weight of approximately 181.62 g/mol. The compound features a chloro group at the 4-position and a methyl group at the 8-position of the quinazoline ring, which contributes to its unique reactivity and biological properties.
Anticancer Properties
Research indicates that this compound exhibits significant anticancer activity . It has been studied for its ability to inhibit cell proliferation and induce apoptosis in various cancer cell lines. The compound's mechanism of action often involves:
- Inhibition of Tyrosine Kinases : These enzymes play a crucial role in cell signaling pathways related to cancer progression. By inhibiting these kinases, this compound disrupts signaling pathways that promote tumor growth and survival .
- Cell Cycle Arrest : The compound can induce cell cycle arrest, particularly at the G2/M phase, leading to reduced proliferation rates in cancer cells .
Case Studies and Research Findings
- In Vitro Studies : A study evaluated the antiproliferative effects of this compound against several tumor cell lines, including HCT-116 (human colorectal carcinoma) and T98G (human glioblastoma). Compounds derived from this base showed promising IC50 values, indicating effective inhibition of cancer cell growth .
- Mechanistic Insights : The compound's ability to bind specifically to key enzymes involved in cancer progression has been documented. This binding leads to a reduction in enzyme activity, which is crucial for understanding its therapeutic potential .
Antimicrobial Activity
In addition to its anticancer effects, this compound has demonstrated antimicrobial properties . Its efficacy against various bacterial strains has been investigated, showcasing potential as an antimicrobial agent.
The antimicrobial activity is attributed to:
- Disruption of Bacterial Cell Wall Synthesis : The compound interferes with metabolic processes critical for bacterial survival .
- Targeting Cytochrome bd Oxidase : Recent studies have shown that quinazoline derivatives can inhibit cytochrome bd oxidase in Mycobacterium tuberculosis, highlighting their potential as antituberculosis agents .
Comparative Analysis with Similar Compounds
The biological activities of this compound can be compared with other quinazoline derivatives:
Compound | Anticancer Activity | Antimicrobial Activity | IC50 (Cancer Cells) |
---|---|---|---|
This compound | High | Moderate | Varies by derivative |
4-Chloro-6-methoxyquinazoline | Moderate | High | ~10 µM |
4-Methylquinazoline | Low | Moderate | >50 µM |
Scientific Research Applications
Chemical Properties and Structure
4-Chloro-8-methylquinazoline has the molecular formula and a molecular weight of approximately 179.62 g/mol. Its structure features:
- Chloro group at the 4-position
- Methyl group at the 8-position
This unique substitution pattern enhances its reactivity and biological properties, making it a valuable subject for drug design and development.
Medicinal Chemistry
This compound has been studied for its potential as an anticancer agent . Research indicates that quinazoline derivatives can inhibit the activity of specific enzymes involved in cancer progression, particularly tyrosine kinases. These kinases play a critical role in cell signaling pathways related to cancer cell growth and survival.
- Case Study : A study demonstrated that quinazoline derivatives exhibit significant anticancer properties by inducing apoptosis in various cancer cell lines. The compound showed effectiveness against breast cancer cells through molecular docking analyses that revealed strong binding affinities to cancer-related targets .
Antimicrobial Activity
The compound has also been evaluated for its antimicrobial properties . It has shown effectiveness against various bacterial strains, suggesting its potential application in treating bacterial infections.
- Mechanism of Action : The antimicrobial activity may involve disruption of bacterial cell wall synthesis and interference with metabolic processes essential for bacterial growth. In vitro assays indicated that similar compounds displayed significant antimicrobial activity against common pathogens .
Material Science
In addition to its biological applications, this compound serves as a building block for synthesizing more complex quinazoline derivatives. This is particularly useful in developing new materials and chemical processes within industrial settings.
Case Studies and Research Findings
- Anticancer Studies : Research on substituted quinazolines found varying binding affinities to cancer-related targets, indicating their potential as therapeutic agents against breast cancer .
- Antimicrobial Efficacy : In vitro assays demonstrated that compounds similar to this compound exhibited significant antimicrobial activity against methicillin-resistant Staphylococcus aureus (MRSA), highlighting its application in treating resistant infections .
- Pharmacokinetic Properties : Studies suggest that quinazoline derivatives comply with Lipinski's Rule of Five, indicating favorable absorption and permeability characteristics essential for drug development .
Q & A
Q. Basic: What are the common synthetic methodologies for preparing 4-Chloro-8-methylquinazoline, and how do they compare in efficiency?
This compound is typically synthesized via cyclization or halogenation of quinazoline precursors. Traditional methods include coupling substituted benzyl chlorides with 2-aminobenzamides under high temperatures (≥100°C) using transition metal catalysts . Electrochemical methods, such as oxidative cyclization in undivided cells with aluminum/carbon electrodes and acetic acid electrolytes, offer milder conditions (room temperature) and higher yields (up to 85%) compared to thermal approaches . Key metrics for comparison include reaction time, purity, and scalability.
Q. Advanced: How can electrochemical synthesis parameters be optimized to minimize byproducts in this compound production?
Electrode material, current density, and electrolyte composition critically influence byproduct formation. For instance, carbon electrodes paired with acetic acid reduce oxidation side reactions compared to metal electrodes. A stepwise optimization protocol involves:
Screening electrolytes (e.g., acetic acid vs. sulfuric acid) for protonation efficiency.
Adjusting current density (0.5–2.0 mA/cm²) to balance reaction rate and selectivity.
Monitoring reaction progress via HPLC to identify intermediates .
Studies show that lower current densities (0.8 mA/cm²) improve selectivity for the chloro-substituted product .
Q. Basic: What spectroscopic techniques are essential for characterizing this compound?
Core techniques include:
- ¹H/¹³C NMR : To confirm the quinazoline backbone and substituent positions (e.g., methyl at C8, chloro at C4).
- Mass Spectrometry (HRMS) : For molecular ion validation (e.g., [M+H]⁺ at m/z 193.04).
- IR Spectroscopy : To detect C-Cl stretches (~550–600 cm⁻¹) and C=N vibrations (~1600 cm⁻¹) .
Cross-referencing with databases like SpectraBase ensures accurate peak assignments .
Q. Advanced: How can researchers resolve spectral ambiguities in this compound derivatives?
Overlapping signals in NMR (e.g., aromatic protons) can be addressed via:
2D NMR (COSY, HSQC) : To correlate coupled protons and assign substituent positions.
Deuterated Solvent Screening : DMSO-d₆ may resolve proton splitting better than CDCl₃.
Computational Modeling : DFT calculations (e.g., B3LYP/6-31G*) predict chemical shifts, aiding assignments . For mass spectrometry, isotopic patterns (e.g., chlorine’s M+2 peak) confirm halogen presence .
Q. Basic: What safety protocols are critical when handling this compound?
- PPE : Impervious gloves (e.g., nitrile), sealed goggles, and lab coats .
- Ventilation : Use fume hoods to avoid inhalation.
- Decontamination : Ethanol/water mixtures (70:30) for spills; avoid skin contact .
- Storage : In airtight containers under inert gas (N₂/Ar) to prevent hydrolysis .
Q. Advanced: How should researchers mitigate risks during exothermic reactions involving this compound?
- Reaction Calorimetry : Monitor heat flow to detect runaway reactions.
- Inert Atmosphere : Use Schlenk lines for moisture-sensitive steps.
- Emergency Quenching : Pre-cooled (−20°C) ethanol/ice baths to halt uncontrolled exotherms . Post-reaction, validate decontamination via TLC to ensure no residual reactive intermediates .
Q. Basic: How do substituents on the quinazoline core influence biological activity?
The methyl group at C8 enhances lipophilicity, improving membrane permeability, while the chloro at C4 increases electrophilicity, aiding covalent interactions with biological targets (e.g., kinase inhibitors) . SAR studies show that replacing C4-Cl with bulkier groups (e.g., Br, CF₃) reduces potency, underscoring steric limitations .
Q. Advanced: What rational design strategies optimize this compound for targeted drug discovery?
Bioisosteric Replacement : Substitute C4-Cl with boronic acid for protease inhibition.
Scaffold Hopping : Integrate the quinazoline core into polycyclic systems (e.g., pyrimidoquinazolines) to modulate selectivity .
Molecular Dynamics : Simulate binding affinities with kinase domains (e.g., EGFR) to guide substitutions .
Q. Basic: What statistical methods are recommended for analyzing biological data involving this compound?
- Dose-Response Analysis : Non-linear regression (e.g., GraphPad Prism) to calculate IC₅₀/EC₅₀.
- Error Propagation : Report SEM ± 95% confidence intervals for triplicate assays .
- Outlier Detection : Grubbs’ test (α=0.05) to exclude anomalous data points .
Q. Advanced: How can contradictory data in SAR studies of this compound derivatives be resolved?
Meta-Analysis : Pool data from multiple studies to identify trends (e.g., consistent potency loss with C8-methyl removal) .
Crystallography : Resolve binding mode discrepancies via X-ray structures of ligand-target complexes .
Machine Learning : Train QSAR models on diverse datasets to predict outliers . Transparent reporting of solvent effects and assay conditions (e.g., buffer pH) is critical .
Comparison with Similar Compounds
Comparison with Structurally Similar Compounds
Substituent Effects on Reactivity and Bioactivity
2,4-Dichloro-8-methylquinazoline (CAS 5190-68-1)
- Structural Difference : Additional chlorine at position 2.
- Impact: The second chlorine reduces inhibitory activity in kinase assays compared to mono-chloro derivatives. For example, compound 8l (2,4-dichloro-substituted) showed ~50% lower activity than 8k (4-chloro-8-methyl) in enzymatic assays .
4-Chloro-8-fluoro-7-methoxyquinazoline (CAS 1934560-94-7)
- Structural Differences : Fluorine at position 8 and methoxy at position 5.
- Impact :
4-Chloro-2,6-dimethylquinazoline (CAS 39576-83-5)
- Structural Difference : Methyl groups at positions 2 and 4.
- Impact: Steric hindrance from methyl groups reduces reactivity in nucleophilic substitutions. Improved inhibitory activity in some contexts; e.g., 3- and 6-methyl derivatives (8e, 8g) showed 2–3× higher activity than mono-methyl analogs .
Physicochemical Properties
Compound | CAS | Molecular Formula | Molecular Weight (g/mol) | XLogP3 | Key Substituents |
---|---|---|---|---|---|
This compound | 58421-80-0 | C₉H₇ClN₂ | 178.62 | 2.9 | Cl (C4), CH₃ (C8) |
2,4-Dichloro-8-methylquinazoline | 5190-68-1 | C₉H₆Cl₂N₂ | 213.06 | 3.5 | Cl (C2, C4), CH₃ (C8) |
4-Chloro-8-fluoro-7-methoxyquinazoline | 1934560-94-7 | C₉H₆ClFN₂O | 212.61 | 2.2 | Cl (C4), F (C8), OCH₃ (C7) |
8-Chloro-2-methyl-4-quinazolone | 19407-54-6 | C₉H₇ClN₂O | 194.62 | 1.8 | Cl (C8), CH₃ (C2), ketone (C4) |
Key Observations :
- Lipophilicity decreases with polar groups (e.g., methoxy in 1934560-94-7: XLogP3 = 2.2 vs. 2.9 in 58421-80-0).
- Halogenation (Cl, F) enhances electrophilic reactivity, critical for cross-coupling reactions .
Preparation Methods
Phosphorus Oxychloride (POCl₃)-Mediated Chlorination
Phosphorus oxychloride is the most widely employed reagent for converting 8-methylquinazolin-4(3H)-one to 4-chloro-8-methylquinazoline. This method leverages POCl₃’s ability to act as both a solvent and a chlorinating agent under controlled conditions.
High-Yield Protocol Under Inert Atmosphere
In a optimized procedure, 8-methylquinazolin-4(3H)-one (125 g, 6.92 mmol) is suspended in POCl₃ (800 mL) under nitrogen atmosphere and refluxed at 120°C for 12 hours. The reaction progress is monitored via TLC and LCMS. Subsequent workup involves evaporating excess POCl₃, dissolving the residue in dichloromethane (DCM), and quenching with ice-cold saturated potassium carbonate. Organic layers are washed with brine, dried over sodium sulfate, and concentrated to yield this compound as a yellow solid (120 g, 86%). This method prioritizes scalability and reproducibility, making it suitable for industrial applications.
Key Data:
Yield | Reaction Conditions | Key Steps |
---|---|---|
86% | POCl₃, 120°C, 12h, inert atmosphere | Reflux in POCl₃ → Quench with K₂CO₃ → DCM extraction → Drying → Concentration |
Acetonitrile-Based Reflux Method
A smaller-scale variant uses acetonitrile (ACN) as the solvent. Here, 8-methylquinazolin-4(3H)-one (1.1 g) is dissolved in ACN (155 mL), followed by POCl₃ (10.1 mL). The mixture is refluxed until completion, cooled, and purified via silica gel chromatography (ethyl acetate/hexane). While this approach avoids excess POCl₃, the yield drops to 35%, likely due to incomplete chlorination or side reactions.
Key Data:
Yield | Reaction Conditions | Key Steps |
---|---|---|
35% | POCl₃, ACN, reflux | Reflux in ACN → Solvent removal → Column purification |
Thionyl Chloride (SOCl₂)-Driven Chlorination
Thionyl chloride offers an alternative route, particularly when paired with catalytic dimethylformamide (DMF). This method is notable for shorter reaction times and simplified workup.
DMF-Catalyzed Protocol
A mixture of 8-methylquinazolin-4(3H)-one (20 g) and DMF (0.1 mL) in SOCl₂ (50 mL) is refluxed overnight. After cooling, excess SOCl₂ is removed under vacuum, and the residue is neutralized with saturated sodium carbonate. Extraction with DCM and chromatography (petroleum ether/ethyl acetate) yields this compound (1.96 g, 88%). The use of DMF enhances chlorination efficiency by activating the carbonyl group.
Key Data:
Yield | Reaction Conditions | Key Steps |
---|---|---|
88% | SOCl₂, DMF, reflux, 12h | Reflux with DMF → Neutralization → DCM extraction |
Solvent-Free Adaptation
In solvent-free conditions, 8-methylquinazolin-4(3H)-one is heated directly with SOCl₂ (27.4 mL) and DMF (2 drops) at reflux for 8 hours. Post-reaction processing mirrors the DMF-catalyzed method, yielding 65.1–88.9% product. This variant reduces solvent waste but requires careful temperature control to prevent decomposition.
Comparative Analysis of Methodologies
Yield and Efficiency
- POCl₃ (Inert Atmosphere): Maximum yield (86%) with industrial scalability but demands rigorous inert conditions.
- POCl₃ (ACN): Lower yield (35%) due to solvent interference but suitable for lab-scale synthesis.
- SOCl₂ (DMF): High yield (88%) with shorter reaction times, ideal for rapid production.
Practical Considerations
- Safety: POCl₃ reactions require nitrogen purging to mitigate hydrolytic side reactions, whereas SOCl₂ releases HCl gas, necessitating fume hood use.
- Purification: POCl₃ methods often bypass chromatography, while SOCl₂ routes may require it for optimal purity.
- Cost: SOCl₂ is more cost-effective for small batches, whereas bulk POCl₃ usage is economically viable for large-scale synthesis.
Mechanistic Insights
Chlorination proceeds via nucleophilic acyl substitution. POCl₃ phosphorylates the carbonyl oxygen, rendering the C4 position electrophilic for chloride attack. Similarly, SOCl₂ converts the hydroxyl group into a better leaving group (chlorosulfite intermediate), facilitating chloride substitution. The methyl group at C8 exerts minimal steric hindrance, ensuring regioselectivity.
Properties
IUPAC Name |
4-chloro-8-methylquinazoline | |
---|---|---|
Source | PubChem | |
URL | https://pubchem.ncbi.nlm.nih.gov | |
Description | Data deposited in or computed by PubChem | |
InChI |
InChI=1S/C9H7ClN2/c1-6-3-2-4-7-8(6)11-5-12-9(7)10/h2-5H,1H3 | |
Source | PubChem | |
URL | https://pubchem.ncbi.nlm.nih.gov | |
Description | Data deposited in or computed by PubChem | |
InChI Key |
UTBDPFFXKANFFT-UHFFFAOYSA-N | |
Source | PubChem | |
URL | https://pubchem.ncbi.nlm.nih.gov | |
Description | Data deposited in or computed by PubChem | |
Canonical SMILES |
CC1=C2C(=CC=C1)C(=NC=N2)Cl | |
Source | PubChem | |
URL | https://pubchem.ncbi.nlm.nih.gov | |
Description | Data deposited in or computed by PubChem | |
Molecular Formula |
C9H7ClN2 | |
Source | PubChem | |
URL | https://pubchem.ncbi.nlm.nih.gov | |
Description | Data deposited in or computed by PubChem | |
DSSTOX Substance ID |
DTXSID50592667 | |
Record name | 4-Chloro-8-methylquinazoline | |
Source | EPA DSSTox | |
URL | https://comptox.epa.gov/dashboard/DTXSID50592667 | |
Description | DSSTox provides a high quality public chemistry resource for supporting improved predictive toxicology. | |
Molecular Weight |
178.62 g/mol | |
Source | PubChem | |
URL | https://pubchem.ncbi.nlm.nih.gov | |
Description | Data deposited in or computed by PubChem | |
CAS No. |
58421-80-0 | |
Record name | 4-Chloro-8-methylquinazoline | |
Source | CAS Common Chemistry | |
URL | https://commonchemistry.cas.org/detail?cas_rn=58421-80-0 | |
Description | CAS Common Chemistry is an open community resource for accessing chemical information. Nearly 500,000 chemical substances from CAS REGISTRY cover areas of community interest, including common and frequently regulated chemicals, and those relevant to high school and undergraduate chemistry classes. This chemical information, curated by our expert scientists, is provided in alignment with our mission as a division of the American Chemical Society. | |
Explanation | The data from CAS Common Chemistry is provided under a CC-BY-NC 4.0 license, unless otherwise stated. | |
Record name | 4-Chloro-8-methylquinazoline | |
Source | EPA DSSTox | |
URL | https://comptox.epa.gov/dashboard/DTXSID50592667 | |
Description | DSSTox provides a high quality public chemistry resource for supporting improved predictive toxicology. | |
Record name | 4-chloro-8-methylquinazoline | |
Source | European Chemicals Agency (ECHA) | |
URL | https://echa.europa.eu/information-on-chemicals | |
Description | The European Chemicals Agency (ECHA) is an agency of the European Union which is the driving force among regulatory authorities in implementing the EU's groundbreaking chemicals legislation for the benefit of human health and the environment as well as for innovation and competitiveness. | |
Explanation | Use of the information, documents and data from the ECHA website is subject to the terms and conditions of this Legal Notice, and subject to other binding limitations provided for under applicable law, the information, documents and data made available on the ECHA website may be reproduced, distributed and/or used, totally or in part, for non-commercial purposes provided that ECHA is acknowledged as the source: "Source: European Chemicals Agency, http://echa.europa.eu/". Such acknowledgement must be included in each copy of the material. ECHA permits and encourages organisations and individuals to create links to the ECHA website under the following cumulative conditions: Links can only be made to webpages that provide a link to the Legal Notice page. | |
Synthesis routes and methods I
Procedure details
Synthesis routes and methods II
Procedure details
Synthesis routes and methods III
Procedure details
Retrosynthesis Analysis
AI-Powered Synthesis Planning: Our tool employs the Template_relevance Pistachio, Template_relevance Bkms_metabolic, Template_relevance Pistachio_ringbreaker, Template_relevance Reaxys, Template_relevance Reaxys_biocatalysis model, leveraging a vast database of chemical reactions to predict feasible synthetic routes.
One-Step Synthesis Focus: Specifically designed for one-step synthesis, it provides concise and direct routes for your target compounds, streamlining the synthesis process.
Accurate Predictions: Utilizing the extensive PISTACHIO, BKMS_METABOLIC, PISTACHIO_RINGBREAKER, REAXYS, REAXYS_BIOCATALYSIS database, our tool offers high-accuracy predictions, reflecting the latest in chemical research and data.
Strategy Settings
Precursor scoring | Relevance Heuristic |
---|---|
Min. plausibility | 0.01 |
Model | Template_relevance |
Template Set | Pistachio/Bkms_metabolic/Pistachio_ringbreaker/Reaxys/Reaxys_biocatalysis |
Top-N result to add to graph | 6 |
Feasible Synthetic Routes
Disclaimer and Information on In-Vitro Research Products
Please be aware that all articles and product information presented on BenchChem are intended solely for informational purposes. The products available for purchase on BenchChem are specifically designed for in-vitro studies, which are conducted outside of living organisms. In-vitro studies, derived from the Latin term "in glass," involve experiments performed in controlled laboratory settings using cells or tissues. It is important to note that these products are not categorized as medicines or drugs, and they have not received approval from the FDA for the prevention, treatment, or cure of any medical condition, ailment, or disease. We must emphasize that any form of bodily introduction of these products into humans or animals is strictly prohibited by law. It is essential to adhere to these guidelines to ensure compliance with legal and ethical standards in research and experimentation.