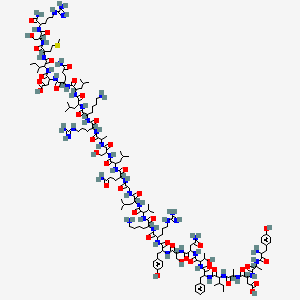
Sermorelin
Overview
Description
Sermorelin is a synthetic peptide that mimics the action of the naturally occurring growth hormone-releasing hormone. It consists of the first 29 amino acids of the endogenous growth hormone-releasing hormone, making it the shortest synthetic peptide that retains full biological activity. This compound is primarily used to stimulate the production and release of growth hormone from the pituitary gland, which has various applications in medicine and research .
Preparation Methods
Synthetic Routes and Reaction Conditions
Sermorelin is synthesized through solid-phase peptide synthesis, a method that allows the sequential addition of amino acids to a growing peptide chain. The process involves the following steps:
Attachment of the first amino acid: The first amino acid is attached to a solid resin support.
Deprotection and coupling: The amino group of the attached amino acid is deprotected, and the next amino acid is coupled to it using a coupling reagent such as dicyclohexylcarbodiimide.
Repetition: The deprotection and coupling steps are repeated until the desired peptide sequence is obtained.
Cleavage and purification: The peptide is cleaved from the resin and purified using high-performance liquid chromatography
Industrial Production Methods
In industrial settings, the production of this compound involves large-scale solid-phase peptide synthesis. The process is automated to ensure consistency and efficiency. The synthesized peptide is then subjected to rigorous quality control measures, including mass spectrometry and amino acid analysis, to confirm its purity and identity .
Chemical Reactions Analysis
Types of Reactions
Sermorelin can undergo various chemical reactions, including:
Oxidation: The methionine residue in this compound can be oxidized to methionine sulfoxide.
Reduction: Disulfide bonds, if present, can be reduced to free thiols.
Substitution: Amino acid residues in this compound can be substituted with other amino acids to create analogs with different properties
Common Reagents and Conditions
Oxidation: Hydrogen peroxide or other oxidizing agents.
Reduction: Dithiothreitol or other reducing agents.
Substitution: Amino acid derivatives and coupling reagents
Major Products Formed
Oxidation: Methionine sulfoxide.
Reduction: Free thiols from disulfide bonds.
Substitution: Peptide analogs with modified amino acid sequences
Scientific Research Applications
Sermorelin has a wide range of scientific research applications, including:
Medicine: Used to diagnose and treat growth hormone deficiency in children and adults. .
Biology: Used to study the regulation of growth hormone secretion and the effects of growth hormone on various physiological processes
Chemistry: Employed in the synthesis of peptide analogs and the study of peptide chemistry
Industry: Used in the development of peptide-based therapeutics and diagnostic agents
Mechanism of Action
Sermorelin exerts its effects by binding to the growth hormone-releasing hormone receptor on the anterior pituitary gland. This binding stimulates the release of growth hormone, which then acts on various tissues in the body to promote growth and metabolism. The primary molecular target of this compound is the growth hormone-releasing hormone receptor, and the pathway involved includes the activation of adenylate cyclase and the subsequent increase in cyclic adenosine monophosphate levels .
Comparison with Similar Compounds
Similar Compounds
Tesamorelin: Another synthetic peptide that stimulates the release of growth hormone. .
CJC-1295: A synthetic peptide that also stimulates growth hormone release but has a longer half-life compared to sermorelin
Uniqueness of this compound
This compound is unique in its shorter peptide sequence, which makes it the shortest synthetic peptide that retains full biological activity. This property allows for more precise control over its effects and reduces the risk of side effects compared to longer peptides .
Properties
IUPAC Name |
4-[[1-[[1-[[1-[[1-[[4-amino-1-[[1-[[1-[[1-[[6-amino-1-[[1-[[1-[[2-[[5-amino-1-[[1-[[1-[[1-[[1-[[6-amino-1-[[1-[[1-[[5-amino-1-[[1-[[1-[[1-[[1-[(1-amino-5-carbamimidamido-1-oxopentan-2-yl)amino]-3-hydroxy-1-oxopropan-2-yl]amino]-4-methylsulfanyl-1-oxobutan-2-yl]amino]-3-methyl-1-oxopentan-2-yl]amino]-3-carboxy-1-oxopropan-2-yl]amino]-1,5-dioxopentan-2-yl]amino]-4-methyl-1-oxopentan-2-yl]amino]-4-methyl-1-oxopentan-2-yl]amino]-1-oxohexan-2-yl]amino]-5-carbamimidamido-1-oxopentan-2-yl]amino]-1-oxopropan-2-yl]amino]-3-hydroxy-1-oxopropan-2-yl]amino]-4-methyl-1-oxopentan-2-yl]amino]-1,5-dioxopentan-2-yl]amino]-2-oxoethyl]amino]-4-methyl-1-oxopentan-2-yl]amino]-3-methyl-1-oxobutan-2-yl]amino]-1-oxohexan-2-yl]amino]-5-carbamimidamido-1-oxopentan-2-yl]amino]-3-(4-hydroxyphenyl)-1-oxopropan-2-yl]amino]-3-hydroxy-1-oxopropan-2-yl]amino]-1,4-dioxobutan-2-yl]amino]-3-hydroxy-1-oxobutan-2-yl]amino]-1-oxo-3-phenylpropan-2-yl]amino]-3-methyl-1-oxopentan-2-yl]amino]-1-oxopropan-2-yl]amino]-3-[2-[[2-amino-3-(4-hydroxyphenyl)propanoyl]amino]propanoylamino]-4-oxobutanoic acid | |
---|---|---|
Details | Computed by Lexichem TK 2.7.0 (PubChem release 2021.05.07) | |
Source | PubChem | |
URL | https://pubchem.ncbi.nlm.nih.gov | |
Description | Data deposited in or computed by PubChem | |
InChI |
InChI=1S/C149H246N44O42S/c1-20-77(13)116(191-122(211)81(17)168-132(221)104(66-113(204)205)178-121(210)79(15)167-123(212)88(152)62-84-39-43-86(198)44-40-84)145(234)185-102(63-83-32-23-22-24-33-83)138(227)193-118(82(18)197)146(235)186-103(65-111(155)202)137(226)189-108(71-196)142(231)182-101(64-85-41-45-87(199)46-42-85)136(225)175-93(38-31-56-165-149(161)162)126(215)174-91(35-26-28-53-151)131(220)190-115(76(11)12)143(232)184-97(58-72(3)4)124(213)166-68-112(203)170-94(47-49-109(153)200)128(217)180-100(61-75(9)10)135(224)188-106(69-194)140(229)169-80(16)120(209)172-92(37-30-55-164-148(159)160)125(214)173-90(34-25-27-52-150)127(216)179-99(60-74(7)8)134(223)181-98(59-73(5)6)133(222)176-95(48-50-110(154)201)129(218)183-105(67-114(206)207)139(228)192-117(78(14)21-2)144(233)177-96(51-57-236-19)130(219)187-107(70-195)141(230)171-89(119(156)208)36-29-54-163-147(157)158/h22-24,32-33,39-46,72-82,88-108,115-118,194-199H,20-21,25-31,34-38,47-71,150-152H2,1-19H3,(H2,153,200)(H2,154,201)(H2,155,202)(H2,156,208)(H,166,213)(H,167,212)(H,168,221)(H,169,229)(H,170,203)(H,171,230)(H,172,209)(H,173,214)(H,174,215)(H,175,225)(H,176,222)(H,177,233)(H,178,210)(H,179,216)(H,180,217)(H,181,223)(H,182,231)(H,183,218)(H,184,232)(H,185,234)(H,186,235)(H,187,219)(H,188,224)(H,189,226)(H,190,220)(H,191,211)(H,192,228)(H,193,227)(H,204,205)(H,206,207)(H4,157,158,163)(H4,159,160,164)(H4,161,162,165) | |
Details | Computed by InChI 1.0.6 (PubChem release 2021.05.07) | |
Source | PubChem | |
URL | https://pubchem.ncbi.nlm.nih.gov | |
Description | Data deposited in or computed by PubChem | |
InChI Key |
WGWPRVFKDLAUQJ-UHFFFAOYSA-N | |
Details | Computed by InChI 1.0.6 (PubChem release 2021.05.07) | |
Source | PubChem | |
URL | https://pubchem.ncbi.nlm.nih.gov | |
Description | Data deposited in or computed by PubChem | |
Canonical SMILES |
CCC(C)C(C(=O)NC(CC1=CC=CC=C1)C(=O)NC(C(C)O)C(=O)NC(CC(=O)N)C(=O)NC(CO)C(=O)NC(CC2=CC=C(C=C2)O)C(=O)NC(CCCNC(=N)N)C(=O)NC(CCCCN)C(=O)NC(C(C)C)C(=O)NC(CC(C)C)C(=O)NCC(=O)NC(CCC(=O)N)C(=O)NC(CC(C)C)C(=O)NC(CO)C(=O)NC(C)C(=O)NC(CCCNC(=N)N)C(=O)NC(CCCCN)C(=O)NC(CC(C)C)C(=O)NC(CC(C)C)C(=O)NC(CCC(=O)N)C(=O)NC(CC(=O)O)C(=O)NC(C(C)CC)C(=O)NC(CCSC)C(=O)NC(CO)C(=O)NC(CCCNC(=N)N)C(=O)N)NC(=O)C(C)NC(=O)C(CC(=O)O)NC(=O)C(C)NC(=O)C(CC3=CC=C(C=C3)O)N | |
Details | Computed by OEChem 2.3.0 (PubChem release 2021.05.07) | |
Source | PubChem | |
URL | https://pubchem.ncbi.nlm.nih.gov | |
Description | Data deposited in or computed by PubChem | |
Molecular Formula |
C149H246N44O42S | |
Details | Computed by PubChem 2.1 (PubChem release 2021.05.07) | |
Source | PubChem | |
URL | https://pubchem.ncbi.nlm.nih.gov | |
Description | Data deposited in or computed by PubChem | |
Molecular Weight |
3357.9 g/mol | |
Details | Computed by PubChem 2.1 (PubChem release 2021.05.07) | |
Source | PubChem | |
URL | https://pubchem.ncbi.nlm.nih.gov | |
Description | Data deposited in or computed by PubChem | |
Mechanism of Action |
Sermorelin binds to the growth hormone releasing hormone receptor and mimics native GRF in its ability to stimulate growth hormone secretion. | |
Record name | Sermorelin | |
Source | DrugBank | |
URL | https://www.drugbank.ca/drugs/DB00010 | |
Description | The DrugBank database is a unique bioinformatics and cheminformatics resource that combines detailed drug (i.e. chemical, pharmacological and pharmaceutical) data with comprehensive drug target (i.e. sequence, structure, and pathway) information. | |
Explanation | Creative Common's Attribution-NonCommercial 4.0 International License (http://creativecommons.org/licenses/by-nc/4.0/legalcode) | |
CAS No. |
86168-78-7 | |
Record name | Sermorelin | |
Source | DrugBank | |
URL | https://www.drugbank.ca/drugs/DB00010 | |
Description | The DrugBank database is a unique bioinformatics and cheminformatics resource that combines detailed drug (i.e. chemical, pharmacological and pharmaceutical) data with comprehensive drug target (i.e. sequence, structure, and pathway) information. | |
Explanation | Creative Common's Attribution-NonCommercial 4.0 International License (http://creativecommons.org/licenses/by-nc/4.0/legalcode) | |
Disclaimer and Information on In-Vitro Research Products
Please be aware that all articles and product information presented on BenchChem are intended solely for informational purposes. The products available for purchase on BenchChem are specifically designed for in-vitro studies, which are conducted outside of living organisms. In-vitro studies, derived from the Latin term "in glass," involve experiments performed in controlled laboratory settings using cells or tissues. It is important to note that these products are not categorized as medicines or drugs, and they have not received approval from the FDA for the prevention, treatment, or cure of any medical condition, ailment, or disease. We must emphasize that any form of bodily introduction of these products into humans or animals is strictly prohibited by law. It is essential to adhere to these guidelines to ensure compliance with legal and ethical standards in research and experimentation.