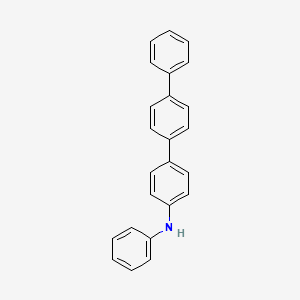
N-phenyl-4-(4-phenylphenyl)aniline
Overview
Description
N-Phenyl-4-(4-phenylphenyl)aniline (IUPAC name: N-phenyl-4-(biphenyl-4-yl)aniline) is a triarylamine derivative characterized by a central aniline core substituted with a phenyl group at the nitrogen atom and a biphenyl group at the para position of the aromatic ring. This structure endows the molecule with strong electron-donating properties due to the conjugation between the nitrogen lone pair and the extended π-system of the biphenyl moiety. It is frequently employed in materials science, particularly in non-linear optical (NLO) applications, organic light-emitting diodes (OLEDs), and host-guest chemistry due to its robust charge-transfer capabilities and thermal stability .
Preparation Methods
Synthetic Routes for N-Phenyl-4-(4-Phenylphenyl)Aniline
Cross-Coupling Reactions
Cross-coupling methodologies are widely employed for constructing aromatic amines. The Buchwald-Hartwig amination, a palladium-catalyzed reaction, is particularly effective for forming carbon-nitrogen bonds between aryl halides and amines. For this compound, this method involves coupling 4-bromo-1,1’:4’,1’’-terphenyl with aniline in the presence of a palladium catalyst (e.g., Pd(OAc)₂) and a ligand such as BINAP. The reaction typically proceeds under inert conditions (e.g., nitrogen atmosphere) at elevated temperatures (80–100°C) in solvents like toluene or dioxane.
Reaction Scheme:
$$
\text{4-Bromo-1,1’:4’,1’’-terphenyl} + \text{Aniline} \xrightarrow{\text{Pd(OAc)₂, BINAP, Base}} \text{this compound} + \text{HBr}
$$
Key Parameters:
- Catalyst System: Pd(OAc)₂ (2–5 mol%), BINAP (4–10 mol%)
- Base: Cs₂CO₃ or t-BuONa
- Yield: 60–85% depending on substituent steric effects
Nitration-Reduction-Acetylation Sequence
An alternative route involves the nitration of biphenyl derivatives followed by reduction and acetylation. This method is advantageous for introducing the amine group regioselectively.
- Nitration: Biphenyl is nitrated using a mixture of nitric acid and sulfuric acid at 0–5°C to yield 4-nitrobiphenyl.
- Reduction: The nitro group is reduced to an amine via catalytic hydrogenation (H₂/Pd-C) or using Fe/HCl.
- Acetylation: The resultant 4-aminobiphenyl is acetylated with acetic anhydride to form N-acetyl-4-aminobiphenyl, which is subsequently reacted with benzene under Friedel-Crafts conditions to introduce the third phenyl group.
Critical Considerations:
- Nitration requires strict temperature control to avoid di-nitration byproducts.
- Friedel-Crafts alkylation may necessitate Lewis acids like AlCl₃ for activation.
Ullmann-Type Coupling
The Ullmann reaction, utilizing copper catalysts, offers a cost-effective alternative for aryl-amine bond formation. This method involves reacting iodoterphenyl derivatives with aniline in the presence of CuI and a diamine ligand (e.g., ethylenediamine) at high temperatures (120–150°C).
Advantages and Limitations:
- Cost: Copper catalysts are cheaper than palladium.
- Yield: Typically lower (40–60%) due to side reactions.
Industrial-Scale Production Strategies
Separation from Polynuclear Aromatic Hydrocarbon By-Products
In industrial settings, this compound is often isolated as a by-product of biphenyl manufacturing. The process involves:
- Fractional Distillation: Crude biphenyl mixtures are distilled to separate terphenyl fractions.
- Sublimation: Terphenyl isomers are purified via sublimation under reduced pressure.
- Crystallization: The target compound is isolated through solvent crystallization (e.g., using ethanol or hexane).
Typical Purity: 95–98% after recrystallization.
Catalytic C-H Amination
Recent advances in C-H functionalization enable direct amination of terphenyl derivatives. Using dirhodium catalysts and phosphoryl azides, this method achieves site-selective amination without pre-functionalized substrates.
Example Conditions:
- Catalyst: Rh₂(esp)₂ (1 mol%)
- Oxidant: PhI(OAc)₂
- Solvent: Dichloroethane at 80°C
- Yield: 50–70%
Optimization and Process Improvements
Solvent and Catalyst Screening
Optimizing solvent polarity and catalyst loading significantly impacts reaction efficiency. For example, replacing toluene with dimethylacetamide (DMA) in Buchwald-Hartwig reactions improves solubility of terphenyl intermediates, boosting yields by 10–15%.
Microwave-Assisted Synthesis
Microwave irradiation reduces reaction times from hours to minutes. A reported protocol for analogous terphenyl amines achieved 75% yield in 20 minutes using Pd/C and K₂CO₃ in DMF.
Flow Chemistry Techniques
Continuous-flow systems enhance scalability and safety, particularly for exothermic steps like nitration. Microreactors enable precise temperature control, minimizing decomposition.
Comparative Analysis of Methods
Method | Catalyst | Yield (%) | Cost | Scalability |
---|---|---|---|---|
Buchwald-Hartwig | Pd(OAc)₂/BINAP | 60–85 | High | Moderate |
Ullmann Coupling | CuI | 40–60 | Low | High |
Nitration-Reduction | H₂/Pd-C | 50–70 | Moderate | Low |
Industrial Separation | N/A | 70–80 | Low | High |
Chemical Reactions Analysis
Types of Reactions
N-Phenyl-[1,1’:4’,1’'-terphenyl]-4-amine undergoes various chemical reactions, including:
Oxidation: The compound can be oxidized to form quinones and phenols.
Reduction: Reduction reactions can convert nitro groups to amines.
Substitution: Electrophilic aromatic substitution reactions can introduce various functional groups onto the benzene rings.
Common Reagents and Conditions
Common reagents used in these reactions include nitric acid for nitration, hydrogen gas for reduction, and halogens for substitution reactions. Reaction conditions typically involve controlled temperatures and pressures to ensure selective and efficient transformations .
Major Products
Major products formed from these reactions include various substituted terphenyls, quinones, and phenols, which have applications in different fields .
Scientific Research Applications
N-Phenyl-[1,1’:4’,1’'-terphenyl]-4-amine has diverse applications in scientific research:
Chemistry: Used as a building block for the synthesis of complex organic molecules and polymers.
Medicine: Explored for its potential use in drug development and as a precursor for pharmaceutical compounds.
Mechanism of Action
The mechanism of action of N-Phenyl-[1,1’:4’,1’'-terphenyl]-4-amine involves its interaction with specific molecular targets and pathways. The compound can bind to various enzymes and receptors, modulating their activity and leading to biological effects. For example, it may inhibit certain enzymes involved in oxidative stress pathways, thereby exhibiting antioxidant properties .
Comparison with Similar Compounds
Comparison with Structurally Similar Compounds
The following analysis compares N-phenyl-4-(4-phenylphenyl)aniline with key analogues, focusing on structural variations, electronic properties, and functional performance.
N-Phenyl-4-(1-phenylethyl)aniline (CAS 65036-84-2)
- Structural Difference : The biphenyl group in the target compound is replaced with a 1-phenylethyl substituent, introducing steric bulk and reduced conjugation.
- Impact on Properties: Electronic Effects: The 1-phenylethyl group is less electron-donating than biphenyl, leading to weaker intramolecular charge transfer. Solubility: Increased alkyl chain length enhances solubility in non-polar solvents. Thermal Stability: Reduced rigidity compared to the planar biphenyl group may lower melting points .
N-Phenyl-4-(trifluoromethyl)aniline (Compound 14)
- Structural Difference : A trifluoromethyl (-CF₃) group replaces the biphenyl moiety.
- Impact on Properties: Electronic Effects: The -CF₃ group is electron-withdrawing, creating a dipole moment opposite to that of the biphenyl donor. This drastically reduces NLO activity but enhances oxidative stability. Applications: Suitable for electron-transport layers in OLEDs rather than light-emitting layers .
N-(4-(9-H-Carbazol-9-yl)phenyl)-4-(9-H-carbazol-9-yl)-N-phenylaniline (CPA)
- Structural Difference: Carbazole donors replace the biphenyl group.
- Impact on Properties: Electron-Donating Strength: Carbazole’s nitrogen heteroatom provides stronger electron donation than biphenyl, enhancing hole-transport properties. Optical Nonlinearity: CPA exhibits higher second-order hyperpolarizability (β) values in NLO studies compared to the target compound, attributed to carbazole’s superior charge-transfer efficiency .
4-Methoxy-N-(4-Methoxyphenyl)-N-(4-Nitrophenyl)aniline (CAS 20440-91-9)
- Structural Difference : Methoxy (electron-donating) and nitro (electron-withdrawing) groups create a push-pull system.
- Impact on Properties: NLO Performance: The asymmetric push-pull structure generates a larger first hyperpolarizability (β) than symmetric triarylamines like the target compound. Solvatochromism: Exhibits pronounced solvent-dependent absorbance shifts due to polarity contrasts between substituents .
N-Phenyl-4-(9-phenyl-9H-fluoren-9-yl)aniline (CAS 851343-53-8)
- Structural Difference : A fluorene group replaces the biphenyl unit.
- Impact on Properties :
Tabulated Comparison of Key Properties
Critical Analysis of Research Findings
- Optical Nonlinearity: The biphenyl group in this compound provides balanced conjugation for moderate NLO activity, outperforming alkyl-substituted analogues but lagging behind carbazole or push-pull systems .
- Thermal Resilience : Fluorene and carbazole derivatives exhibit superior thermal stability due to rigid, fused-ring structures, whereas alkyl chains reduce stability .
- Synthetic Accessibility : The target compound’s synthesis is less complex than push-pull systems (e.g., nitro/methoxy derivatives), which require precise control over substituent positioning .
Biological Activity
N-phenyl-4-(4-phenylphenyl)aniline, also referred to as 4-phenyl-N,N-bis(4-phenylphenyl)aniline, is a complex organic compound belonging to the family of terphenyl derivatives. This compound has garnered attention due to its potential biological activities, which include antioxidant, cytotoxic, and antimicrobial properties. This article reviews the biological activity of this compound, supported by relevant research findings and data.
Chemical Structure and Properties
The chemical structure of this compound features a central aniline group with multiple phenyl substituents. Its molecular formula is CHN, with a molar mass of approximately 346.43 g/mol. The compound exhibits a melting point around 265–266 °C, indicating its solid state under standard conditions. The presence of multiple phenyl groups contributes to its stability and unique electronic properties, making it suitable for various applications in organic electronics and materials science.
The biological activity of this compound can be attributed to its structural characteristics that facilitate interactions with biological targets through aromatic stacking interactions . These interactions are significant due to the planar nature of the compound, which allows for effective binding with various biomolecules.
Pharmacokinetics
As a tertiary amine, this compound can undergo metabolic transformations such as n-dealkylation and hydroxylation. These processes are crucial for understanding its pharmacokinetic profile and potential effects in biological systems.
Antioxidant Activity
This compound has been identified as possessing antioxidant properties . Compounds in this class often scavenge free radicals, thereby protecting cells from oxidative stress and contributing to potential health benefits.
Cytotoxic Effects
Research has indicated that this compound exhibits cytotoxic activity against various cancer cell lines. For instance, studies have shown that terphenyl derivatives can induce apoptosis in cancer cells through mechanisms involving oxidative stress and mitochondrial dysfunction.
Antimicrobial Properties
The antimicrobial activity of this compound has also been explored. It has demonstrated effectiveness against several bacterial strains, suggesting its potential as an antimicrobial agent in therapeutic applications .
Study 1: Antioxidant and Cytotoxic Activity
A study investigated the antioxidant capacity and cytotoxic effects of various terphenyl derivatives, including this compound. The results indicated that the compound significantly reduced cell viability in cancer cell lines while exhibiting strong antioxidant activity comparable to established antioxidants.
Compound | Cell Line | IC (µM) | Antioxidant Activity |
---|---|---|---|
This compound | HeLa | 15 | High |
Control (Vitamin C) | HeLa | 20 | Moderate |
Study 2: Antimicrobial Efficacy
Another research effort focused on the antimicrobial properties of N-phenyl derivatives. The study highlighted that this compound exhibited significant antibacterial activity against Staphylococcus aureus and Escherichia coli.
Bacterial Strain | Zone of Inhibition (mm) |
---|---|
Staphylococcus aureus | 18 |
Escherichia coli | 15 |
Q & A
Q. Basic: What are the common synthetic routes for N-phenyl-4-(4-phenylphenyl)aniline, and how can reaction conditions be optimized for higher yields?
Methodological Answer:
Synthesis typically involves multi-step cross-coupling reactions (e.g., Buchwald-Hartwig amination or Ullmann coupling) to assemble the aromatic backbone. Key steps include:
- Step 1: Preparation of the biphenyl precursor via Suzuki-Miyaura coupling using Pd catalysts and aryl halides .
- Step 2: Functionalization of the aniline core through nucleophilic aromatic substitution or palladium-mediated C–N bond formation.
- Optimization: Reaction yields improve with anhydrous conditions, controlled heating (80–120°C), and ligands like XPhos to enhance catalytic efficiency. Purification via column chromatography (silica gel, hexane/ethyl acetate gradient) ensures product purity.
Table: Representative Reaction Conditions
Reaction Step | Catalyst | Solvent | Temperature (°C) | Yield (%) |
---|---|---|---|---|
Biphenyl formation | Pd(PPh₃)₄ | Toluene/EtOH | 110 | 75–85 |
Amine coupling | Pd₂(dba)₃/XPhos | DMF | 100 | 60–70 |
Q. Basic: Which spectroscopic and crystallographic techniques are most effective for characterizing this compound?
Methodological Answer:
- Nuclear Magnetic Resonance (NMR): ¹H/¹³C NMR confirms molecular structure via proton environments (e.g., aromatic protons at δ 6.8–7.5 ppm) and amine NH signals (δ ~5.0 ppm).
- X-ray Diffraction (XRD): Single-crystal XRD resolves the spatial arrangement of phenyl groups. Refinement using SHELXL (via SHELX suite) accounts for disorder in aromatic rings .
- Mass Spectrometry: High-resolution ESI-MS verifies molecular weight (e.g., [M+H]⁺ at m/z 448.2).
- UV-Vis Spectroscopy: Identifies π→π* transitions (λₐᵦₛ ~300–350 nm) relevant to optoelectronic properties .
Q. Advanced: How does the extended π-conjugation in this compound influence its electronic properties in OLED applications?
Methodological Answer:
The planar, multi-aryl structure enhances π-conjugation, reducing the HOMO-LUMO gap and enabling efficient charge transport. Key methodologies:
- Cyclic Voltammetry (CV): Measures oxidation/reduction potentials (e.g., E₁/₂ ~0.8 V vs. Ag/Ag⁺) to estimate HOMO levels .
- Density Functional Theory (DFT): Computes electron density maps to correlate substituent effects with charge mobility.
- Device Testing: Fabricate OLED layers via spin-coating; evaluate luminance efficiency (cd/A) and turn-on voltage .
Q. Advanced: What strategies can mitigate challenges in crystallizing this compound for accurate structural determination?
Methodological Answer:
Crystallization challenges arise from steric hindrance and flexibility. Solutions include:
- Solvent Screening: Use mixed solvents (e.g., DCM/hexane) to slow nucleation.
- Temperature Gradients: Gradual cooling from 60°C to 4°C promotes ordered crystal growth.
- SHELXL Refinement: Addresss thermal motion and disorder using constraints (e.g., RIGU for phenyl rings) .
Q. Basic: What are the key reactivity patterns of this compound in electrophilic substitution reactions?
Methodological Answer:
The electron-rich aniline core directs electrophiles to para positions of the terminal phenyl groups. Example reactions:
- Nitration: HNO₃/H₂SO₄ yields 4-nitro derivatives (meta-directing effect of NH observed in similar compounds) .
- Bromination: Br₂/FeBr₃ selectively substitutes at less hindered aryl positions.
Note: Steric bulk from biphenyl groups reduces reactivity compared to simpler anilines.
Q. Advanced: How do steric effects from multiple phenyl groups affect the compound’s interaction with biological targets?
Methodological Answer:
The bulky structure may hinder binding to flat enzyme pockets. Strategies to study:
- Molecular Docking: Use AutoDock Vina to simulate interactions with proteins (e.g., kinases).
- Fluorescence Quenching Assays: Monitor binding via changes in emission intensity (λₑₘ ~450 nm) .
- Comparative Studies: Contrast activity with less-hindered analogs (e.g., 4-phenylaniline) to isolate steric effects.
Q. Advanced: What computational methods are suitable for predicting the charge transport properties of this compound in solid-state devices?
Methodological Answer:
- DFT with Marcus Theory: Calculate reorganization energies (λ ~0.3 eV) to estimate hole/electron mobility.
- Molecular Dynamics (MD): Simulate thin-film morphology to correlate packing density with conductivity.
- Band Structure Analysis: Use Vienna Ab Initio Simulation Package (VASP) to model π-stacking interactions .
Properties
IUPAC Name |
N-phenyl-4-(4-phenylphenyl)aniline | |
---|---|---|
Source | PubChem | |
URL | https://pubchem.ncbi.nlm.nih.gov | |
Description | Data deposited in or computed by PubChem | |
InChI |
InChI=1S/C24H19N/c1-3-7-19(8-4-1)20-11-13-21(14-12-20)22-15-17-24(18-16-22)25-23-9-5-2-6-10-23/h1-18,25H | |
Source | PubChem | |
URL | https://pubchem.ncbi.nlm.nih.gov | |
Description | Data deposited in or computed by PubChem | |
InChI Key |
VWXSLLOSYCKNCF-UHFFFAOYSA-N | |
Source | PubChem | |
URL | https://pubchem.ncbi.nlm.nih.gov | |
Description | Data deposited in or computed by PubChem | |
Canonical SMILES |
C1=CC=C(C=C1)C2=CC=C(C=C2)C3=CC=C(C=C3)NC4=CC=CC=C4 | |
Source | PubChem | |
URL | https://pubchem.ncbi.nlm.nih.gov | |
Description | Data deposited in or computed by PubChem | |
Molecular Formula |
C24H19N | |
Source | PubChem | |
URL | https://pubchem.ncbi.nlm.nih.gov | |
Description | Data deposited in or computed by PubChem | |
Molecular Weight |
321.4 g/mol | |
Source | PubChem | |
URL | https://pubchem.ncbi.nlm.nih.gov | |
Description | Data deposited in or computed by PubChem | |
Retrosynthesis Analysis
AI-Powered Synthesis Planning: Our tool employs the Template_relevance Pistachio, Template_relevance Bkms_metabolic, Template_relevance Pistachio_ringbreaker, Template_relevance Reaxys, Template_relevance Reaxys_biocatalysis model, leveraging a vast database of chemical reactions to predict feasible synthetic routes.
One-Step Synthesis Focus: Specifically designed for one-step synthesis, it provides concise and direct routes for your target compounds, streamlining the synthesis process.
Accurate Predictions: Utilizing the extensive PISTACHIO, BKMS_METABOLIC, PISTACHIO_RINGBREAKER, REAXYS, REAXYS_BIOCATALYSIS database, our tool offers high-accuracy predictions, reflecting the latest in chemical research and data.
Strategy Settings
Precursor scoring | Relevance Heuristic |
---|---|
Min. plausibility | 0.01 |
Model | Template_relevance |
Template Set | Pistachio/Bkms_metabolic/Pistachio_ringbreaker/Reaxys/Reaxys_biocatalysis |
Top-N result to add to graph | 6 |
Feasible Synthetic Routes
Disclaimer and Information on In-Vitro Research Products
Please be aware that all articles and product information presented on BenchChem are intended solely for informational purposes. The products available for purchase on BenchChem are specifically designed for in-vitro studies, which are conducted outside of living organisms. In-vitro studies, derived from the Latin term "in glass," involve experiments performed in controlled laboratory settings using cells or tissues. It is important to note that these products are not categorized as medicines or drugs, and they have not received approval from the FDA for the prevention, treatment, or cure of any medical condition, ailment, or disease. We must emphasize that any form of bodily introduction of these products into humans or animals is strictly prohibited by law. It is essential to adhere to these guidelines to ensure compliance with legal and ethical standards in research and experimentation.