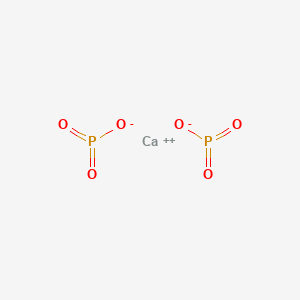
Calcium metaphosphate
Overview
Description
Calcium metaphosphate, also known as calcium pyrophosphate dihydrate (CPPD) is a type of inorganic phosphate that is found naturally in the body. It is composed of calcium, phosphorus, and oxygen atoms, and is found in the bones, teeth, and other tissues. Calcium metaphosphate is important for the structural integrity of bones and teeth, and also plays a role in the biochemical processes that occur in the body. It is an important component of the body's metabolic processes and has been studied extensively for its role in various medical conditions.
Scientific Research Applications
Biomedical Applications
Calcium metaphosphate (CMP) shows significant promise in biomedical applications. It has been used in the preparation of porous ceramics with a calcium metaphosphate fiber skeleton for biomedical use, demonstrating high strength and a large porosity of over 60% (Kasuga et al., 1995). These materials are beneficial due to their biocompatibility and ability to convert into new calcium phosphate phases, such as apatite. Another study explored high-strength β-calcium metaphosphate fibers for biomedical fields, extracted from crystallized products of calcium ultraphosphate glasses, with the potential for various medical applications (Kasuga, Ichino & Abe, 1992).
Bone Graft and Tissue Engineering
Calcium metaphosphate has been evaluated for its role in bone grafts and tissue engineering. A study examined a calcium metaphosphate bone graft containing bone morphogenetic protein-7 in rabbit maxillary defects, finding enhanced bone formation and good biocompatibility (Buranawat et al., 2014). Additionally, the cellular biocompatibility and stimulatory effects of calcium metaphosphate on osteoblastic differentiation of human bone marrow-derived stromal cells were investigated, showing that CMP does not exert a cytotoxic effect and can stimulate osteoblastic differentiation (Park et al., 2004).
Dental Applications
In the dental field, calcium metaphosphate has been used in various applications. The evaluation of calcium metaphosphate-coated implant fixtures compared to conventional resorbable blasted media (RBM) processed implant fixtures found that CMP-coated implants were not inferior to conventional RBM implants, indicating their potential for dental applications (Cho et al., 2013). Another study on filler systems based on calcium metaphosphates explored their potential as fillers for visible-light-activated dental composites, finding that these novel fillers could be useful in resin-based materials such as dental composites, cements, and adhesives (Antonucci, Fowler & Venz, 1991).
properties
InChI |
InChI=1S/Ca.2HO3P/c;2*1-4(2)3/h;2*(H,1,2,3)/q+2;;/p-2 | |
---|---|---|
Source | PubChem | |
URL | https://pubchem.ncbi.nlm.nih.gov | |
Description | Data deposited in or computed by PubChem | |
InChI Key |
ROPDWRCJTIRLTR-UHFFFAOYSA-L | |
Source | PubChem | |
URL | https://pubchem.ncbi.nlm.nih.gov | |
Description | Data deposited in or computed by PubChem | |
Canonical SMILES |
[O-]P(=O)=O.[O-]P(=O)=O.[Ca+2] | |
Source | PubChem | |
URL | https://pubchem.ncbi.nlm.nih.gov | |
Description | Data deposited in or computed by PubChem | |
Molecular Formula |
CaO6P2 | |
Source | PubChem | |
URL | https://pubchem.ncbi.nlm.nih.gov | |
Description | Data deposited in or computed by PubChem | |
DSSTOX Substance ID |
DTXSID10872573 | |
Record name | Calcium bis(metaphosphate) | |
Source | EPA DSSTox | |
URL | https://comptox.epa.gov/dashboard/DTXSID10872573 | |
Description | DSSTox provides a high quality public chemistry resource for supporting improved predictive toxicology. | |
Molecular Weight |
198.02 g/mol | |
Source | PubChem | |
URL | https://pubchem.ncbi.nlm.nih.gov | |
Description | Data deposited in or computed by PubChem | |
Physical Description |
Odourless, colourless crystals or white powder | |
Record name | CALCIUM POLYPHOSPHATE | |
Source | EU Food Improvement Agents | |
URL | https://eur-lex.europa.eu/legal-content/EN/ALL/?uri=CELEX%3A32012R0231 | |
Description | Commission Regulation (EU) No 231/2012 of 9 March 2012 laying down specifications for food additives listed in Annexes II and III to Regulation (EC) No 1333/2008 of the European Parliament and of the Council Text with EEA relevance | |
Solubility |
Usually sparingly soluble in water. Soluble in acid medium | |
Record name | CALCIUM POLYPHOSPHATE | |
Source | EU Food Improvement Agents | |
URL | https://eur-lex.europa.eu/legal-content/EN/ALL/?uri=CELEX%3A32012R0231 | |
Description | Commission Regulation (EU) No 231/2012 of 9 March 2012 laying down specifications for food additives listed in Annexes II and III to Regulation (EC) No 1333/2008 of the European Parliament and of the Council Text with EEA relevance | |
Product Name |
Calcium metaphosphate | |
CAS RN |
13477-39-9, 53801-86-8, 123093-85-6 | |
Record name | Metaphosphoric acid (HPO3), calcium salt (2:1) | |
Source | ChemIDplus | |
URL | https://pubchem.ncbi.nlm.nih.gov/substance/?source=chemidplus&sourceid=0013477399 | |
Description | ChemIDplus is a free, web search system that provides access to the structure and nomenclature authority files used for the identification of chemical substances cited in National Library of Medicine (NLM) databases, including the TOXNET system. | |
Record name | Metaphosphoric acid, calcium salt | |
Source | ChemIDplus | |
URL | https://pubchem.ncbi.nlm.nih.gov/substance/?source=chemidplus&sourceid=0053801868 | |
Description | ChemIDplus is a free, web search system that provides access to the structure and nomenclature authority files used for the identification of chemical substances cited in National Library of Medicine (NLM) databases, including the TOXNET system. | |
Record name | Calcium metaphosphate | |
Source | ChemIDplus | |
URL | https://pubchem.ncbi.nlm.nih.gov/substance/?source=chemidplus&sourceid=0123093856 | |
Description | ChemIDplus is a free, web search system that provides access to the structure and nomenclature authority files used for the identification of chemical substances cited in National Library of Medicine (NLM) databases, including the TOXNET system. | |
Record name | Metaphosphoric acid (HPO3), calcium salt (2:1) | |
Source | EPA Chemicals under the TSCA | |
URL | https://www.epa.gov/chemicals-under-tsca | |
Description | EPA Chemicals under the Toxic Substances Control Act (TSCA) collection contains information on chemicals and their regulations under TSCA, including non-confidential content from the TSCA Chemical Substance Inventory and Chemical Data Reporting. | |
Record name | Calcium bis(metaphosphate) | |
Source | EPA DSSTox | |
URL | https://comptox.epa.gov/dashboard/DTXSID10872573 | |
Description | DSSTox provides a high quality public chemistry resource for supporting improved predictive toxicology. | |
Record name | Calcium bis(metaphosphate) | |
Source | European Chemicals Agency (ECHA) | |
URL | https://echa.europa.eu/substance-information/-/substanceinfo/100.033.412 | |
Description | The European Chemicals Agency (ECHA) is an agency of the European Union which is the driving force among regulatory authorities in implementing the EU's groundbreaking chemicals legislation for the benefit of human health and the environment as well as for innovation and competitiveness. | |
Explanation | Use of the information, documents and data from the ECHA website is subject to the terms and conditions of this Legal Notice, and subject to other binding limitations provided for under applicable law, the information, documents and data made available on the ECHA website may be reproduced, distributed and/or used, totally or in part, for non-commercial purposes provided that ECHA is acknowledged as the source: "Source: European Chemicals Agency, http://echa.europa.eu/". Such acknowledgement must be included in each copy of the material. ECHA permits and encourages organisations and individuals to create links to the ECHA website under the following cumulative conditions: Links can only be made to webpages that provide a link to the Legal Notice page. | |
Synthesis routes and methods I
Procedure details
Synthesis routes and methods II
Procedure details
Disclaimer and Information on In-Vitro Research Products
Please be aware that all articles and product information presented on BenchChem are intended solely for informational purposes. The products available for purchase on BenchChem are specifically designed for in-vitro studies, which are conducted outside of living organisms. In-vitro studies, derived from the Latin term "in glass," involve experiments performed in controlled laboratory settings using cells or tissues. It is important to note that these products are not categorized as medicines or drugs, and they have not received approval from the FDA for the prevention, treatment, or cure of any medical condition, ailment, or disease. We must emphasize that any form of bodily introduction of these products into humans or animals is strictly prohibited by law. It is essential to adhere to these guidelines to ensure compliance with legal and ethical standards in research and experimentation.