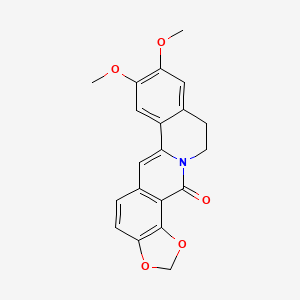
Oxyepiberberine
Overview
Description
Oxyepiberberine is an alkaloid isolated from the medicinal plant Coptis chinensis Franch., commonly known as Huanglian. This compound has garnered significant attention due to its potent biological activities, particularly its anti-cancer properties. This compound has been identified as a novel tubulin polymerization inhibitor, making it a promising candidate for cancer therapy .
Mechanism of Action
Target of Action
Oxyepiberberine, an alkaloid isolated from Coptis chinensis Franch., has been identified to target tubulin . Tubulin is a basic cell component involved in maintaining cell morphology and a variety of cellular processes, including signaling pathways, intracellular macromolecular assembly, and cell division .
Mode of Action
This compound acts as a potent tubulin polymerization inhibitor . It interacts with tubulin, hampering the dynamics of tubulin polymerization, which is highly significant for anti-tumor therapy . This interaction leads to the arrest of the cell cycle and induces apoptosis .
Biochemical Pathways
The primary biochemical pathway affected by this compound is the tubulin polymerization process . By inhibiting this process, this compound disrupts the normal functioning of microtubules, which play a crucial role in cell division . This disruption can lead to cell cycle arrest and apoptosis . Additionally, this compound has been found to regulate SRC-related pathways .
Pharmacokinetics
It has been demonstrated that this compound exhibits potent anti-proliferative effects on ls-1034 human colon cancer cells with an ic50 value of 136 μM . This suggests that this compound may have good bioavailability and effective therapeutic concentrations can be achieved in the body.
Result of Action
This compound has been shown to have potent anti-proliferative effects on various cancer cell lines . It induces apoptosis and inhibits migration of LS-1034 cells in a concentration-dependent manner . In a xenograft mouse model of colon cancer, this compound was able to suppress tumor growth without obvious toxicity .
Action Environment
Biochemical Analysis
Biochemical Properties
Oxyepiberberine has been identified as a potent tubulin polymerization inhibitor . It interacts with tubulin, a globular protein, and inhibits its polymerization, thereby affecting the dynamics of microtubules . This interaction plays a crucial role in its anti-cancer effects.
Cellular Effects
This compound has shown significant anti-proliferative effects on LS-1034 human colon cancer cells . It induces apoptosis and inhibits the migration of these cells in a concentration-dependent manner . These effects influence cell function, including cell signaling pathways and cellular metabolism.
Molecular Mechanism
The molecular mechanism of this compound involves its binding to tubulin, inhibiting its polymerization . This interaction disrupts the dynamics of microtubules, leading to the inhibition of cell proliferation, induction of apoptosis, and reduction in cell migration .
Temporal Effects in Laboratory Settings
The effects of this compound have been observed over time in laboratory settings. It has shown consistent anti-proliferative effects on LS-1034 cells, with its potency remaining stable over the duration of the experiments .
Dosage Effects in Animal Models
In animal models, this compound has demonstrated the ability to suppress tumor growth without obvious toxicity
Preparation Methods
Synthetic Routes and Reaction Conditions: The synthesis of oxyepiberberine typically involves the extraction and isolation from the rhizomes of Coptis chinensis Franch. The process includes several steps:
Extraction: The plant material is subjected to solvent extraction using methanol or ethanol.
Isolation: The crude extract is then subjected to chromatographic techniques such as high-performance liquid chromatography (HPLC) to isolate this compound.
Purification: Further purification is achieved through recrystallization or additional chromatographic methods.
Industrial Production Methods: Industrial production of this compound follows similar extraction and isolation techniques but on a larger scale. The use of advanced chromatographic systems and automated extraction processes ensures higher yields and purity of the compound.
Chemical Reactions Analysis
Types of Reactions: Oxyepiberberine undergoes various chemical reactions, including:
Oxidation: This reaction involves the addition of oxygen or the removal of hydrogen, often using oxidizing agents like hydrogen peroxide or potassium permanganate.
Reduction: The compound can be reduced using reducing agents such as sodium borohydride or lithium aluminum hydride.
Substitution: this compound can undergo nucleophilic or electrophilic substitution reactions, where functional groups are replaced by other groups.
Common Reagents and Conditions:
Oxidation: Hydrogen peroxide, potassium permanganate.
Reduction: Sodium borohydride, lithium aluminum hydride.
Substitution: Various nucleophiles or electrophiles depending on the desired substitution.
Major Products: The major products formed from these reactions depend on the specific conditions and reagents used. For example, oxidation may yield this compound derivatives with additional oxygen-containing functional groups, while reduction may result in the formation of reduced alkaloid derivatives .
Scientific Research Applications
Oxyepiberberine has a wide range of scientific research applications:
Chemistry: Used as a model compound for studying alkaloid chemistry and reaction mechanisms.
Biology: Investigated for its effects on cellular processes, including apoptosis and cell cycle regulation.
Medicine: Explored as a potential anti-cancer agent due to its ability to inhibit tubulin polymerization and induce apoptosis in cancer cells.
Industry: Potential applications in the development of pharmaceuticals and therapeutic agents
Comparison with Similar Compounds
Berberine: Another alkaloid from Coptis chinensis Franch., known for its broad-spectrum biological activities, including anti-cancer, anti-inflammatory, and antimicrobial properties.
Coptisine: An alkaloid with similar anti-cancer properties but differing in its specific molecular targets and mechanisms of action.
Palmatine: Exhibits anti-inflammatory and antimicrobial activities, with some overlap in anti-cancer effects.
Uniqueness of Oxyepiberberine: this compound stands out due to its potent inhibition of tubulin polymerization, a mechanism not commonly observed in other related alkaloids. This unique action makes it particularly effective against certain types of cancer cells, such as colon cancer cells .
Properties
IUPAC Name |
16,17-dimethoxy-5,7-dioxa-1-azapentacyclo[11.8.0.03,11.04,8.014,19]henicosa-3(11),4(8),9,12,14,16,18-heptaen-2-one | |
---|---|---|
Source | PubChem | |
URL | https://pubchem.ncbi.nlm.nih.gov | |
Description | Data deposited in or computed by PubChem | |
InChI |
InChI=1S/C20H17NO5/c1-23-16-8-11-5-6-21-14(13(11)9-17(16)24-2)7-12-3-4-15-19(26-10-25-15)18(12)20(21)22/h3-4,7-9H,5-6,10H2,1-2H3 | |
Source | PubChem | |
URL | https://pubchem.ncbi.nlm.nih.gov | |
Description | Data deposited in or computed by PubChem | |
InChI Key |
PJTYPIGQKDTERS-UHFFFAOYSA-N | |
Source | PubChem | |
URL | https://pubchem.ncbi.nlm.nih.gov | |
Description | Data deposited in or computed by PubChem | |
Canonical SMILES |
COC1=C(C=C2C(=C1)CCN3C2=CC4=C(C3=O)C5=C(C=C4)OCO5)OC | |
Source | PubChem | |
URL | https://pubchem.ncbi.nlm.nih.gov | |
Description | Data deposited in or computed by PubChem | |
Molecular Formula |
C20H17NO5 | |
Source | PubChem | |
URL | https://pubchem.ncbi.nlm.nih.gov | |
Description | Data deposited in or computed by PubChem | |
Molecular Weight |
351.4 g/mol | |
Source | PubChem | |
URL | https://pubchem.ncbi.nlm.nih.gov | |
Description | Data deposited in or computed by PubChem | |
Retrosynthesis Analysis
AI-Powered Synthesis Planning: Our tool employs the Template_relevance Pistachio, Template_relevance Bkms_metabolic, Template_relevance Pistachio_ringbreaker, Template_relevance Reaxys, Template_relevance Reaxys_biocatalysis model, leveraging a vast database of chemical reactions to predict feasible synthetic routes.
One-Step Synthesis Focus: Specifically designed for one-step synthesis, it provides concise and direct routes for your target compounds, streamlining the synthesis process.
Accurate Predictions: Utilizing the extensive PISTACHIO, BKMS_METABOLIC, PISTACHIO_RINGBREAKER, REAXYS, REAXYS_BIOCATALYSIS database, our tool offers high-accuracy predictions, reflecting the latest in chemical research and data.
Strategy Settings
Precursor scoring | Relevance Heuristic |
---|---|
Min. plausibility | 0.01 |
Model | Template_relevance |
Template Set | Pistachio/Bkms_metabolic/Pistachio_ringbreaker/Reaxys/Reaxys_biocatalysis |
Top-N result to add to graph | 6 |
Feasible Synthetic Routes
Disclaimer and Information on In-Vitro Research Products
Please be aware that all articles and product information presented on BenchChem are intended solely for informational purposes. The products available for purchase on BenchChem are specifically designed for in-vitro studies, which are conducted outside of living organisms. In-vitro studies, derived from the Latin term "in glass," involve experiments performed in controlled laboratory settings using cells or tissues. It is important to note that these products are not categorized as medicines or drugs, and they have not received approval from the FDA for the prevention, treatment, or cure of any medical condition, ailment, or disease. We must emphasize that any form of bodily introduction of these products into humans or animals is strictly prohibited by law. It is essential to adhere to these guidelines to ensure compliance with legal and ethical standards in research and experimentation.