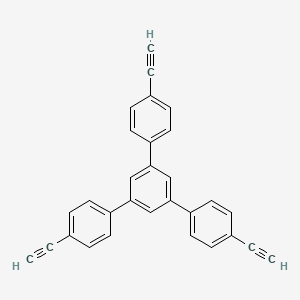
1,3,5-Tris(4-ethynylphenyl)benzene
Overview
Description
1,3,5-Tris(4-ethynylphenyl)benzene is a compound with the molecular formula C30H18. It features a benzene ring substituted with three 4-ethynylphenyl groups at the 1, 3, and 5 positions. This compound is known for its trigonal structure and is commonly used in the synthesis of porous conjugated polymers and semiconducting covalent organic frameworks .
Mechanism of Action
Target of Action
The primary target of 1,3,5-Tris(4-ethynylphenyl)benzene, also known as 4,4’‘-Diethynyl-5’-(4-ethynylphenyl)-1,1’:3’,1’'-terphenyl, is hydrogen peroxide (H2O2) . This compound is used in the synthesis of conjugated polymers, which are becoming increasingly appealing for biological applications .
Mode of Action
This compound interacts with its target through an oxidative coupling reaction . The compound is synthesized into a polymer known as poly(1,3,5-tris(4-ethynylphenyl)-benzene) (PTEPB), a 1,3-diyne-linked conjugated polymer . The electrochemical properties of PTEPB are then measured using hydrogen peroxide as a biomarker .
Biochemical Pathways
The compound affects the electrochemical pathways involved in the detection of hydrogen peroxide . The specific active sites in these pathways are the 1,3-diyne linkages in PTEPB .
Result of Action
The interaction of this compound with hydrogen peroxide results in satisfactory electrochemical responses . This indicates the potential of the compound in the sensitive monitoring of H2O2 released from living cells .
Action Environment
The action, efficacy, and stability of this compound can be influenced by various environmental factors. For instance, the compound’s ability to detect hydrogen peroxide can be affected by the presence of other physiological species . .
Biochemical Analysis
Biochemical Properties
1,3,5-Tris(4-ethynylphenyl)benzene plays a crucial role in various biochemical reactions. It interacts with enzymes, proteins, and other biomolecules, facilitating the formation of complex structures. For instance, it is involved in the synthesis of porous conjugated polymers, which are essential for various biochemical applications . The interactions between this compound and these biomolecules are primarily based on π-π interactions and hydrogen bonding, which contribute to the stability and functionality of the resulting structures .
Cellular Effects
The effects of this compound on various types of cells and cellular processes are profound. This compound influences cell function by modulating cell signaling pathways, gene expression, and cellular metabolism. For example, it has been observed to affect the expression of genes involved in metabolic pathways, thereby altering cellular metabolism . Additionally, this compound can impact cell signaling pathways by interacting with specific proteins and enzymes, leading to changes in cellular responses .
Molecular Mechanism
The molecular mechanism of action of this compound involves several key processes. At the molecular level, this compound exerts its effects through binding interactions with biomolecules, enzyme inhibition or activation, and changes in gene expression. For instance, it can bind to specific enzymes, inhibiting their activity and thereby affecting the biochemical pathways they regulate . Additionally, this compound can modulate gene expression by interacting with transcription factors and other regulatory proteins .
Temporal Effects in Laboratory Settings
In laboratory settings, the effects of this compound change over time. This compound exhibits stability under various conditions, but it can degrade over extended periods, leading to changes in its biochemical activity . Long-term studies have shown that this compound can have lasting effects on cellular function, including alterations in gene expression and metabolic pathways . These temporal effects are crucial for understanding the long-term implications of using this compound in biochemical research.
Dosage Effects in Animal Models
The effects of this compound vary with different dosages in animal models. At lower doses, this compound can enhance certain biochemical pathways, leading to beneficial effects on cellular function . At higher doses, this compound can exhibit toxic or adverse effects, including cellular damage and disruption of metabolic processes . Understanding the dosage effects is essential for optimizing the use of this compound in research and therapeutic applications.
Metabolic Pathways
This compound is involved in several metabolic pathways, interacting with various enzymes and cofactors. This compound can influence metabolic flux and metabolite levels by modulating the activity of key enzymes in these pathways . For example, it has been shown to interact with enzymes involved in the synthesis of conjugated polymers, affecting the overall metabolic processes within cells .
Transport and Distribution
The transport and distribution of this compound within cells and tissues are mediated by specific transporters and binding proteins. This compound can be transported across cell membranes and distributed to various cellular compartments, where it exerts its biochemical effects . The localization and accumulation of this compound within cells are influenced by its interactions with transporters and binding proteins, which facilitate its movement and function .
Subcellular Localization
This compound exhibits specific subcellular localization, which is crucial for its activity and function. This compound can be directed to particular compartments or organelles within cells through targeting signals or post-translational modifications . The subcellular localization of this compound affects its biochemical activity, as it interacts with specific biomolecules within these compartments to exert its effects .
Preparation Methods
Synthetic Routes and Reaction Conditions
1,3,5-Tris(4-ethynylphenyl)benzene can be synthesized through multiple synthetic routes. One common method involves the Sonogashira coupling reaction between multifunctional alkyne-terminal compounds and aromatic iodine compounds. This method avoids the difficulty of separating compounds with similar polarity .
A typical synthetic route includes:
Starting Materials: 1,3,5-tribromobenzene and 3-methylbutynol.
Intermediate Formation: 1,3,5-tris(3’-hydroxy-3’-methyl-but-1’-ynyl)benzene is formed.
Cleavage Reaction: The intermediate undergoes a cleavage reaction in the presence of potassium hydroxide and toluene to form 1,3,5-triethynylbenzene.
Final Coupling: 1,3,5-triethynylbenzene reacts with 4-trimethylsiylethynyl iodobenzene to yield this compound.
Industrial Production Methods
Industrial production methods for this compound typically involve large-scale Sonogashira coupling reactions, optimized for high yield and purity. The reaction conditions are carefully controlled to ensure the efficient formation of the desired product.
Chemical Reactions Analysis
Types of Reactions
1,3,5-Tris(4-ethynylphenyl)benzene undergoes various chemical reactions, including:
Oxidation: The compound can be oxidized to form corresponding quinones.
Reduction: Reduction reactions can yield partially hydrogenated derivatives.
Substitution: Electrophilic substitution reactions can occur on the benzene ring.
Common Reagents and Conditions
Oxidation: Common oxidizing agents include potassium permanganate and chromium trioxide.
Reduction: Hydrogenation reactions typically use palladium or platinum catalysts.
Substitution: Electrophilic substitution reactions often use reagents like bromine or chlorine under acidic conditions.
Major Products
The major products formed from these reactions include quinones, hydrogenated derivatives, and halogenated compounds, depending on the specific reaction conditions and reagents used .
Scientific Research Applications
1,3,5-Tris(4-ethynylphenyl)benzene has a wide range of scientific research applications, including:
Comparison with Similar Compounds
Similar Compounds
1,3,5-Triphenylbenzene: Similar in structure but lacks the ethynyl groups, making it less reactive in certain applications.
1,3,5-Triethynylbenzene: Lacks the phenyl groups, resulting in different electronic properties and reactivity.
Uniqueness
1,3,5-Tris(4-ethynylphenyl)benzene is unique due to its combination of ethynyl and phenyl groups, which provide both extended π-conjugation and structural stability. This makes it particularly useful in the synthesis of advanced materials with high luminescence and selective adsorption properties .
Properties
IUPAC Name |
1,3,5-tris(4-ethynylphenyl)benzene | |
---|---|---|
Source | PubChem | |
URL | https://pubchem.ncbi.nlm.nih.gov | |
Description | Data deposited in or computed by PubChem | |
InChI |
InChI=1S/C30H18/c1-4-22-7-13-25(14-8-22)28-19-29(26-15-9-23(5-2)10-16-26)21-30(20-28)27-17-11-24(6-3)12-18-27/h1-3,7-21H | |
Source | PubChem | |
URL | https://pubchem.ncbi.nlm.nih.gov | |
Description | Data deposited in or computed by PubChem | |
InChI Key |
XJIJQOFZIULKCI-UHFFFAOYSA-N | |
Source | PubChem | |
URL | https://pubchem.ncbi.nlm.nih.gov | |
Description | Data deposited in or computed by PubChem | |
Canonical SMILES |
C#CC1=CC=C(C=C1)C2=CC(=CC(=C2)C3=CC=C(C=C3)C#C)C4=CC=C(C=C4)C#C | |
Source | PubChem | |
URL | https://pubchem.ncbi.nlm.nih.gov | |
Description | Data deposited in or computed by PubChem | |
Molecular Formula |
C30H18 | |
Source | PubChem | |
URL | https://pubchem.ncbi.nlm.nih.gov | |
Description | Data deposited in or computed by PubChem | |
DSSTOX Substance ID |
DTXSID00531521 | |
Record name | 1,3,5-tris(4-ethynylphenyl)benzene | |
Source | EPA DSSTox | |
URL | https://comptox.epa.gov/dashboard/DTXSID00531521 | |
Description | DSSTox provides a high quality public chemistry resource for supporting improved predictive toxicology. | |
Molecular Weight |
378.5 g/mol | |
Source | PubChem | |
URL | https://pubchem.ncbi.nlm.nih.gov | |
Description | Data deposited in or computed by PubChem | |
CAS No. |
71866-86-9 | |
Record name | 1,3,5-tris(4-ethynylphenyl)benzene | |
Source | EPA DSSTox | |
URL | https://comptox.epa.gov/dashboard/DTXSID00531521 | |
Description | DSSTox provides a high quality public chemistry resource for supporting improved predictive toxicology. | |
Retrosynthesis Analysis
AI-Powered Synthesis Planning: Our tool employs the Template_relevance Pistachio, Template_relevance Bkms_metabolic, Template_relevance Pistachio_ringbreaker, Template_relevance Reaxys, Template_relevance Reaxys_biocatalysis model, leveraging a vast database of chemical reactions to predict feasible synthetic routes.
One-Step Synthesis Focus: Specifically designed for one-step synthesis, it provides concise and direct routes for your target compounds, streamlining the synthesis process.
Accurate Predictions: Utilizing the extensive PISTACHIO, BKMS_METABOLIC, PISTACHIO_RINGBREAKER, REAXYS, REAXYS_BIOCATALYSIS database, our tool offers high-accuracy predictions, reflecting the latest in chemical research and data.
Strategy Settings
Precursor scoring | Relevance Heuristic |
---|---|
Min. plausibility | 0.01 |
Model | Template_relevance |
Template Set | Pistachio/Bkms_metabolic/Pistachio_ringbreaker/Reaxys/Reaxys_biocatalysis |
Top-N result to add to graph | 6 |
Feasible Synthetic Routes
Q1: How does TEPB lend itself to the creation of structured materials?
A1: TEPB's structure, with its three symmetrical ethynyl groups, allows it to participate in diverse self-assembly processes. For example, on a silver (Ag(111)) surface, TEPB molecules can form highly ordered, porous honeycomb networks. [, ] This self-assembly is driven by a combination of weak interactions like C–H···π bonding and the molecule's interaction with the substrate. [] Researchers have demonstrated that the resulting two-dimensional networks exhibit organizational chirality and can be further modified through reactions like cyclotrimerization. [, ]
Q2: What makes TEPB interesting for photocatalytic applications?
A2: TEPB can be used as a building block for conjugated microporous polymer nanosheets (CMPNs). These CMPNs exhibit remarkable photocatalytic activity in overall water splitting, efficiently producing stoichiometric amounts of hydrogen (H2) and oxygen (O2) under visible light irradiation. [, ] This capability stems from the polymer's suitable band structure, enabling efficient charge separation and water redox reactions. [] The high surface area provided by the microporous structure further enhances the photocatalytic performance. []
Q3: Can the structure of TEPB-based polymers be modified to influence their properties?
A3: Absolutely! The properties of TEPB-based polymers can be fine-tuned by incorporating other building blocks during synthesis. For instance, oxygen doping during the preparation of carbon nitride aerogel significantly enhances charge separation efficiency and alters the band structure, resulting in a more negative conduction band position and a narrower band gap. [] These modifications significantly improve the material's performance in photocatalytic hydrogen evolution. []
Q4: How do researchers study the mechanisms of TEPB-based photocatalysts?
A4: In situ techniques like diffuse reflectance infrared Fourier transform spectroscopy (DRIFTS) and X-ray photoelectron spectroscopy (XPS) have been instrumental in uncovering the water dissociation pathways on two-dimensional conjugated polymers derived from TEPB. [] These methods allow researchers to identify crucial reaction intermediates and correlate them with computational models. Notably, the studies revealed that water dissociation pathways could differ based on the specific polymer structure, highlighting the importance of structural control in catalyst design. []
Q5: Beyond photocatalysis, are there other applications for TEPB derivatives?
A5: Yes, indeed. TEPB can be utilized to synthesize Poly(1,3,5-tris(4-ethynylphenyl)-benzene) (PTEPB), a conjugated polymer with promising applications in electrochemical sensing. [] Specifically, PTEPB exhibits excellent sensitivity for detecting hydrogen peroxide (H2O2), a crucial biomarker in various biological processes. [] The 1,3-diyne-linkages in PTEPB act as specific active sites for H2O2 detection, as confirmed by control experiments and quantum chemistry calculations. []
Disclaimer and Information on In-Vitro Research Products
Please be aware that all articles and product information presented on BenchChem are intended solely for informational purposes. The products available for purchase on BenchChem are specifically designed for in-vitro studies, which are conducted outside of living organisms. In-vitro studies, derived from the Latin term "in glass," involve experiments performed in controlled laboratory settings using cells or tissues. It is important to note that these products are not categorized as medicines or drugs, and they have not received approval from the FDA for the prevention, treatment, or cure of any medical condition, ailment, or disease. We must emphasize that any form of bodily introduction of these products into humans or animals is strictly prohibited by law. It is essential to adhere to these guidelines to ensure compliance with legal and ethical standards in research and experimentation.