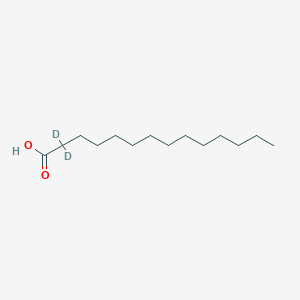
Tetradecanoic-2,2-D2 acid
Overview
Description
Myristic acid is a 14-carbon saturated fatty acid commonly found in animal and vegetable fats, particularly in butterfat, coconut oil, palm oil, and nutmeg oil . The deuterium labeling makes Myristic Acid-d2 useful as an internal standard for the quantification of myristic acid in various analytical applications .
Mechanism of Action
Target of Action
Tetradecanoic-2,2-D2 acid, also known as Myristic acid-d2, is a fatty acid that naturally occurs in some foods such as nutmeg, coconut oil, and palm oil . The primary targets of Myristic acid-d2 are proteins involved in various cellular processes. For instance, it has been found to interact with proteins like Genome polyprotein, cAMP-dependent protein kinase inhibitor alpha, and cAMP-dependent protein kinase catalytic subunit alpha .
Mode of Action
The interaction of this compound with its targets results in various changes within the cell. For instance, it has been found to inhibit the action of carboxylesterase (EC 3.1.1.1), an enzyme involved in the hydrolysis of carboxylic ester compounds . This inhibition can affect the metabolism of certain drugs and other xenobiotics within the body.
Biochemical Pathways
this compound affects various biochemical pathways within the body. For instance, it has been found to play a role in the regulation of autophagy, a cellular process involved in the degradation and recycling of cellular components . It is also involved in the regulation of lipid metabolism, particularly in the synthesis of triglycerides .
Result of Action
The action of this compound at the molecular and cellular level results in various effects. For instance, its role in autophagy regulation can influence cellular health and function . Its involvement in lipid metabolism can affect the levels of triglycerides and other lipids within the body .
Biochemical Analysis
Biochemical Properties
Tetradecanoic-2,2-D2 acid interacts with various enzymes, proteins, and other biomolecules. It is known to inhibit the action of carboxylesterase (EC 3.1.1.1), an enzyme involved in the hydrolysis of carboxylic ester compounds . The nature of these interactions is primarily inhibitory, affecting the enzymatic activity and thus influencing biochemical reactions .
Cellular Effects
The effects of this compound on cells are diverse. It has been found to have anti-virulence properties, reducing the production of pyocyanin, a virulence factor in Pseudomonas aeruginosa, by 35–58% at concentrations of 40 and 1,000 µM . It also reduces swarming by 90% without affecting biofilm formation . These effects indicate that this compound can influence cell function, including impact on cell signaling pathways, gene expression, and cellular metabolism .
Molecular Mechanism
At the molecular level, this compound exerts its effects through binding interactions with biomolecules and changes in gene expression. It is known to inhibit the action of carboxylesterase, an enzyme involved in the hydrolysis of carboxylic ester compounds . This inhibition can lead to changes in the metabolic processes within the cell .
Temporal Effects in Laboratory Settings
It is known that this compound has a molecular weight of 228.3709 , which may influence its stability and degradation over time.
Dosage Effects in Animal Models
In animal models, the coadministration of this compound (200 and 1,000 µM) with Pseudomonas aeruginosa PA14 induced greater damage and reduced survival of the animals up to 50% . This suggests that the effects of this compound can vary with different dosages, and high doses may have toxic or adverse effects .
Metabolic Pathways
This compound is involved in the fatty acid biosynthesis pathway . It interacts with enzymes and cofactors within this pathway, potentially affecting metabolic flux or metabolite levels .
Preparation Methods
Synthetic Routes and Reaction Conditions: Myristic Acid-d2 can be synthesized through the deuteration of myristic acid. This process involves the replacement of hydrogen atoms with deuterium atoms. The deuteration can be achieved using deuterium gas (D2) in the presence of a catalyst under controlled conditions .
Industrial Production Methods: Industrial production of Myristic Acid-d2 typically involves the use of deuterated reagents and solvents to ensure high levels of deuterium incorporation. The process may include steps such as hydrogenation, deuteration, and purification to obtain the final product with high purity and deuterium content .
Chemical Reactions Analysis
Types of Reactions: Myristic Acid-d2 undergoes similar chemical reactions as myristic acid, including:
Oxidation: Myristic Acid-d2 can be oxidized to form myristic aldehyde and myristic alcohol.
Reduction: Reduction of Myristic Acid-d2 can yield myristyl alcohol.
Esterification: Myristic Acid-d2 can react with alcohols to form esters, such as myristyl myristate.
Common Reagents and Conditions:
Oxidation: Common oxidizing agents include potassium permanganate (KMnO4) and chromium trioxide (CrO3).
Reduction: Reducing agents such as lithium aluminum hydride (LiAlH4) or sodium borohydride (NaBH4) are commonly used.
Esterification: Acid catalysts like sulfuric acid (H2SO4) or p-toluenesulfonic acid (PTSA) are used in esterification reactions.
Major Products Formed:
Oxidation: Myristic aldehyde and myristic alcohol.
Reduction: Myristyl alcohol.
Esterification: Myristyl myristate.
Scientific Research Applications
Myristic Acid-d2 has a wide range of applications in scientific research:
Comparison with Similar Compounds
Palmitic Acid-d2: A 16-carbon saturated fatty acid with similar deuterium labeling used for similar analytical purposes.
Stearic Acid-d2: An 18-carbon saturated fatty acid used in lipid research and quantification.
Uniqueness: Myristic Acid-d2 is unique due to its specific 14-carbon chain length and its role in protein lipidation, which is not as prominent in longer-chain fatty acids like palmitic acid and stearic acid .
Properties
IUPAC Name |
2,2-dideuteriotetradecanoic acid | |
---|---|---|
Source | PubChem | |
URL | https://pubchem.ncbi.nlm.nih.gov | |
Description | Data deposited in or computed by PubChem | |
InChI |
InChI=1S/C14H28O2/c1-2-3-4-5-6-7-8-9-10-11-12-13-14(15)16/h2-13H2,1H3,(H,15,16)/i13D2 | |
Source | PubChem | |
URL | https://pubchem.ncbi.nlm.nih.gov | |
Description | Data deposited in or computed by PubChem | |
InChI Key |
TUNFSRHWOTWDNC-KLTYLHELSA-N | |
Source | PubChem | |
URL | https://pubchem.ncbi.nlm.nih.gov | |
Description | Data deposited in or computed by PubChem | |
Canonical SMILES |
CCCCCCCCCCCCCC(=O)O | |
Source | PubChem | |
URL | https://pubchem.ncbi.nlm.nih.gov | |
Description | Data deposited in or computed by PubChem | |
Isomeric SMILES |
[2H]C([2H])(CCCCCCCCCCCC)C(=O)O | |
Source | PubChem | |
URL | https://pubchem.ncbi.nlm.nih.gov | |
Description | Data deposited in or computed by PubChem | |
Molecular Formula |
C14H28O2 | |
Source | PubChem | |
URL | https://pubchem.ncbi.nlm.nih.gov | |
Description | Data deposited in or computed by PubChem | |
Molecular Weight |
230.38 g/mol | |
Source | PubChem | |
URL | https://pubchem.ncbi.nlm.nih.gov | |
Description | Data deposited in or computed by PubChem | |
Synthesis routes and methods
Procedure details
Retrosynthesis Analysis
AI-Powered Synthesis Planning: Our tool employs the Template_relevance Pistachio, Template_relevance Bkms_metabolic, Template_relevance Pistachio_ringbreaker, Template_relevance Reaxys, Template_relevance Reaxys_biocatalysis model, leveraging a vast database of chemical reactions to predict feasible synthetic routes.
One-Step Synthesis Focus: Specifically designed for one-step synthesis, it provides concise and direct routes for your target compounds, streamlining the synthesis process.
Accurate Predictions: Utilizing the extensive PISTACHIO, BKMS_METABOLIC, PISTACHIO_RINGBREAKER, REAXYS, REAXYS_BIOCATALYSIS database, our tool offers high-accuracy predictions, reflecting the latest in chemical research and data.
Strategy Settings
Precursor scoring | Relevance Heuristic |
---|---|
Min. plausibility | 0.01 |
Model | Template_relevance |
Template Set | Pistachio/Bkms_metabolic/Pistachio_ringbreaker/Reaxys/Reaxys_biocatalysis |
Top-N result to add to graph | 6 |
Feasible Synthetic Routes
Disclaimer and Information on In-Vitro Research Products
Please be aware that all articles and product information presented on BenchChem are intended solely for informational purposes. The products available for purchase on BenchChem are specifically designed for in-vitro studies, which are conducted outside of living organisms. In-vitro studies, derived from the Latin term "in glass," involve experiments performed in controlled laboratory settings using cells or tissues. It is important to note that these products are not categorized as medicines or drugs, and they have not received approval from the FDA for the prevention, treatment, or cure of any medical condition, ailment, or disease. We must emphasize that any form of bodily introduction of these products into humans or animals is strictly prohibited by law. It is essential to adhere to these guidelines to ensure compliance with legal and ethical standards in research and experimentation.