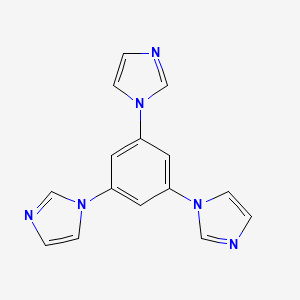
1,3,5-tri(1H-imidazol-1-yl)benzene
Overview
Description
1,3,5-tri(1H-imidazol-1-yl)benzene is an organic compound with the chemical formula C15H12N6. It is a white crystalline solid that is stable at room temperature but may decompose under high temperatures and light exposure . This compound is known for its unique structure, which consists of a benzene ring substituted with three imidazole groups at the 1, 3, and 5 positions.
Mechanism of Action
Target of Action
It has been used as an organic linker in the synthesis of novel metal-organic frameworks (mofs) with al, cr, and cu as metal ions .
Mode of Action
It has been used in the formation of supramolecular columnar liquid crystals through h-bonding .
Result of Action
It has been used in the formation of supramolecular columnar liquid crystals that exhibit strong fluorescence .
Action Environment
It is known to be stable at room temperature but may decompose under high temperature and light exposure .
Preparation Methods
Synthetic Routes and Reaction Conditions
1,3,5-tri(1H-imidazol-1-yl)benzene can be synthesized through various methods. One common synthetic route involves the condensation reaction between benzoyl chloride and 1-imidazolecarboxaldehyde . The reaction typically requires a catalyst and is carried out under controlled temperature and pressure conditions to ensure high yield and purity.
Industrial Production Methods
In an industrial setting, the production of this compound may involve large-scale condensation reactions using automated reactors. The reaction conditions are optimized to maximize efficiency and minimize by-products. The final product is then purified through crystallization or other separation techniques to achieve the desired purity level.
Chemical Reactions Analysis
Types of Reactions
1,3,5-tri(1H-imidazol-1-yl)benzene undergoes various chemical reactions, including:
Oxidation: The compound can be oxidized using strong oxidizing agents, leading to the formation of imidazole derivatives.
Reduction: Reduction reactions can convert the imidazole groups into other functional groups, depending on the reducing agent used.
Common Reagents and Conditions
Oxidation: Common oxidizing agents include potassium permanganate and hydrogen peroxide.
Reduction: Reducing agents such as lithium aluminum hydride or sodium borohydride are often used.
Substitution: Various halogenating agents and nucleophiles can be used for substitution reactions.
Major Products
The major products formed from these reactions depend on the specific reagents and conditions used. For example, oxidation may yield imidazole N-oxides, while reduction can produce imidazole-substituted amines.
Scientific Research Applications
1,3,5-tri(1H-imidazol-1-yl)benzene has a wide range of applications in scientific research:
Biology: The compound’s ability to bind to metal ions makes it useful in studying metalloproteins and other biological systems.
Medicine: Research is ongoing to explore its potential as a therapeutic agent, particularly in drug delivery systems and as a scaffold for designing new pharmaceuticals.
Industry: It is used in the synthesis of advanced materials, including catalysts and sensors.
Comparison with Similar Compounds
Similar Compounds
1,3,5-tris(1-imidazolyl)benzene: Similar in structure but may have different substituents on the imidazole rings.
1,3,5-tris(imidazol-1-ylmethyl)benzene: Contains additional methylene groups linking the imidazole rings to the benzene core.
Uniqueness
1,3,5-tri(1H-imidazol-1-yl)benzene is unique due to its specific substitution pattern and the presence of three imidazole groups directly attached to the benzene ring. This structure provides distinct chemical and physical properties, making it particularly useful in forming stable metal complexes and in various applications in materials science and biochemistry.
Properties
IUPAC Name |
1-[3,5-di(imidazol-1-yl)phenyl]imidazole | |
---|---|---|
Source | PubChem | |
URL | https://pubchem.ncbi.nlm.nih.gov | |
Description | Data deposited in or computed by PubChem | |
InChI |
InChI=1S/C15H12N6/c1-4-19(10-16-1)13-7-14(20-5-2-17-11-20)9-15(8-13)21-6-3-18-12-21/h1-12H | |
Source | PubChem | |
URL | https://pubchem.ncbi.nlm.nih.gov | |
Description | Data deposited in or computed by PubChem | |
InChI Key |
QAPWMCQWTGBLSM-UHFFFAOYSA-N | |
Source | PubChem | |
URL | https://pubchem.ncbi.nlm.nih.gov | |
Description | Data deposited in or computed by PubChem | |
Canonical SMILES |
C1=CN(C=N1)C2=CC(=CC(=C2)N3C=CN=C3)N4C=CN=C4 | |
Source | PubChem | |
URL | https://pubchem.ncbi.nlm.nih.gov | |
Description | Data deposited in or computed by PubChem | |
Molecular Formula |
C15H12N6 | |
Source | PubChem | |
URL | https://pubchem.ncbi.nlm.nih.gov | |
Description | Data deposited in or computed by PubChem | |
Molecular Weight |
276.30 g/mol | |
Source | PubChem | |
URL | https://pubchem.ncbi.nlm.nih.gov | |
Description | Data deposited in or computed by PubChem | |
Retrosynthesis Analysis
AI-Powered Synthesis Planning: Our tool employs the Template_relevance Pistachio, Template_relevance Bkms_metabolic, Template_relevance Pistachio_ringbreaker, Template_relevance Reaxys, Template_relevance Reaxys_biocatalysis model, leveraging a vast database of chemical reactions to predict feasible synthetic routes.
One-Step Synthesis Focus: Specifically designed for one-step synthesis, it provides concise and direct routes for your target compounds, streamlining the synthesis process.
Accurate Predictions: Utilizing the extensive PISTACHIO, BKMS_METABOLIC, PISTACHIO_RINGBREAKER, REAXYS, REAXYS_BIOCATALYSIS database, our tool offers high-accuracy predictions, reflecting the latest in chemical research and data.
Strategy Settings
Precursor scoring | Relevance Heuristic |
---|---|
Min. plausibility | 0.01 |
Model | Template_relevance |
Template Set | Pistachio/Bkms_metabolic/Pistachio_ringbreaker/Reaxys/Reaxys_biocatalysis |
Top-N result to add to graph | 6 |
Feasible Synthetic Routes
Q1: What makes 1,3,5-tri(1H-imidazol-1-yl)benzene attractive for building metal-organic frameworks (MOFs)?
A1: this compound possesses three imidazole rings, acting as nitrogen donors to coordinate with metal ions. [, , , , , , , ] This tridentate nature, coupled with the molecule's relatively rigid structure, allows the creation of robust and porous frameworks with diverse architectures. [, , , , , , , ]
Q2: How does the structure of this compound influence the dimensionality of the resulting MOFs?
A2: The trigonal symmetry and the spatial arrangement of the imidazole groups in tib can lead to the formation of MOFs with varying dimensionality. Researchers have synthesized 1D chains, [] 2D layered structures, [, ] and even complex 3D frameworks [, ] by carefully selecting co-ligands and metal ions, showcasing tib's versatility in constructing diverse MOF architectures.
Q3: Can you provide specific examples of how this compound-based MOFs have been utilized for sensing applications?
A3: A Cd(II)-based MOF incorporating this compound has demonstrated high sensitivity and selectivity for detecting nitroaromatic compounds, especially 2,4,6-trinitrophenol (TNP) and 4-nitrophenol (4-NP) in solution. [] This detection relies on the fluorescence quenching of the MOF upon interaction with the target molecules.
Q4: Beyond sensing, are there other reported applications for this compound in materials chemistry?
A4: Yes, researchers have explored this compound-based MOFs for applications beyond sensing. For instance, a series of multifunctional MOFs incorporating tib exhibited excellent adsorption properties for harmful organic dyes, showing promise for environmental remediation. [] Additionally, a Co-based coordination polymer with tib displayed potential as a photocatalyst for the degradation of organic dyes under UV/Vis light irradiation. []
Q5: Are there any studies investigating the biological activity of this compound-containing compounds?
A5: While research primarily focuses on material science applications, some studies explore the biological activity of tib-containing complexes. For example, a Co-based coordination polymer with this compound showed inhibitory effects on human osteosarcoma cells. [, ] Additionally, trinuclear Pt(II) complexes using tib as a bridging ligand exhibited promising activity as G-quadruplex binders and telomerase inhibitors, suggesting potential anticancer applications. []
Q6: Have theoretical studies been conducted on this compound or its complexes?
A6: Yes, computational methods have been employed to investigate the properties of tib-containing materials. For instance, density functional theory (DFT) calculations were used to analyze the electronic structure and band gap of a Co-based coordination polymer, providing insights into its photocatalytic activity for dye degradation. []
Disclaimer and Information on In-Vitro Research Products
Please be aware that all articles and product information presented on BenchChem are intended solely for informational purposes. The products available for purchase on BenchChem are specifically designed for in-vitro studies, which are conducted outside of living organisms. In-vitro studies, derived from the Latin term "in glass," involve experiments performed in controlled laboratory settings using cells or tissues. It is important to note that these products are not categorized as medicines or drugs, and they have not received approval from the FDA for the prevention, treatment, or cure of any medical condition, ailment, or disease. We must emphasize that any form of bodily introduction of these products into humans or animals is strictly prohibited by law. It is essential to adhere to these guidelines to ensure compliance with legal and ethical standards in research and experimentation.