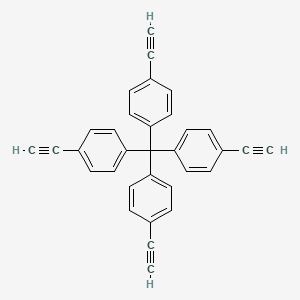
Tetrakis(4-ethynylphenyl)methane
Overview
Description
Tetrakis(4-ethynylphenyl)methane is an organic compound with the molecular formula C33H20. It is a tetrahedral molecule where a central carbon atom is bonded to four ethynylphenyl groups.
Mechanism of Action
Target of Action
Tetrakis(4-ethynylphenyl)methane is a tetraethynyl monomer that is primarily used in the synthesis of microporous organic polymers (MOPs) and covalent organic frameworks (COFs) . Its primary targets are the building blocks of these polymers and frameworks, where it serves as a connector molecule .
Mode of Action
The compound interacts with its targets through a conventional Sonogashira–Hagihara coupling reaction . This reaction allows the formation of complex structures such as MOPs and COFs. The resulting structures are characterized by their high porosity and specific surface area .
Biochemical Pathways
The biochemical pathways affected by this compound are primarily related to the synthesis of MOPs and COFs . These polymers and frameworks have various applications, including efficient adsorption of Lewis acidic CO2 molecules .
Pharmacokinetics
Its physical properties such as solubility and stability are crucial for its function in the synthesis of mops and cofs .
Result of Action
The result of this compound’s action is the formation of MOPs and COFs with high porosity and specific surface area . These structures can exhibit efficient adsorption of Lewis acidic CO2 molecules and display good CO2-over-N2 selectivity .
Action Environment
The synthesis of MOPs and COFs using this compound typically requires an inert gas atmosphere and relatively high reaction temperatures . The compound’s action, efficacy, and stability can be influenced by these and other environmental factors .
Biochemical Analysis
Biochemical Properties
Tetrakis(4-ethynylphenyl)methane is used as a building block in the synthesis of MOPs . These polymers have good porosity and accessible active sites . The incorporation of other moieties into the polymer skeleton, such as triphenylamine or azobenzene, increases the number of electron-donating basic nitrogen sites in the porous frameworks . This allows these MOPs to exhibit efficient adsorption of Lewis acidic CO2 molecules and display good CO2-over-N2 selectivity .
Cellular Effects
The MOPs synthesized using this compound have been shown to efficiently adsorb CO2 molecules This suggests that these polymers could potentially influence cellular processes related to gas transport and exchange
Molecular Mechanism
The molecular mechanism of this compound primarily involves its role in the synthesis of MOPs . These polymers are synthesized via the Sonogashira–Hagihara coupling reaction, a process that involves the formation of carbon-carbon bonds between the ethynyl groups of this compound and other monomers .
Temporal Effects in Laboratory Settings
The MOPs synthesized using this compound have been shown to have good stability and porosity .
Preparation Methods
Synthetic Routes and Reaction Conditions
Tetrakis(4-ethynylphenyl)methane can be synthesized through the Sonogashira–Hagihara coupling reaction. This method involves the coupling of tetrakis(4-iodophenyl)methane with trimethylsilylacetylene in the presence of a palladium catalyst and copper iodide as a co-catalyst. The reaction is typically carried out in an inert atmosphere, such as nitrogen or argon, to prevent oxidation .
Industrial Production Methods
While specific industrial production methods for this compound are not widely documented, the Sonogashira–Hagihara coupling reaction remains a standard approach. The scalability of this method makes it suitable for industrial applications, provided that the reaction conditions are optimized for larger-scale synthesis .
Chemical Reactions Analysis
Types of Reactions
Tetrakis(4-ethynylphenyl)methane undergoes various chemical reactions, including:
Oxidation: The ethynyl groups can be oxidized to form corresponding carbonyl compounds.
Substitution: The ethynyl groups can participate in substitution reactions, such as halogenation or hydrogenation.
Common Reagents and Conditions
Oxidation: Reagents like potassium permanganate or osmium tetroxide can be used under mild conditions.
Substitution: Halogenation can be achieved using bromine or chlorine in the presence of a suitable solvent.
Major Products
Oxidation: The major products are typically carbonyl compounds.
Substitution: Depending on the substituent introduced, various halogenated or hydrogenated derivatives can be formed.
Scientific Research Applications
Tetrakis(4-ethynylphenyl)methane has several applications in scientific research:
Materials Science: It is used in the synthesis of microporous organic polymers (MOPs) which have applications in gas storage and separation.
Organic Chemistry: The compound serves as a building block for the construction of complex molecular architectures.
Biology and Medicine: While direct applications in biology and medicine are limited, its derivatives are explored for potential use in drug delivery systems.
Industry: It is used in the development of advanced materials with specific electronic and optical properties.
Comparison with Similar Compounds
Similar Compounds
Tetrakis(4-ethynylphenyl)silane: Similar in structure but with a silicon atom at the center instead of carbon.
Tetrakis(4-iodophenyl)methane: Precursor to tetrakis(4-ethynylphenyl)methane, with iodine atoms instead of ethynyl groups.
Uniqueness
This compound is unique due to its ability to form highly porous and stable structures, making it particularly useful in materials science applications. Its ethynyl groups provide versatility in chemical reactions, allowing for the synthesis of a wide range of derivatives .
Properties
IUPAC Name |
1-ethynyl-4-[tris(4-ethynylphenyl)methyl]benzene | |
---|---|---|
Source | PubChem | |
URL | https://pubchem.ncbi.nlm.nih.gov | |
Description | Data deposited in or computed by PubChem | |
InChI |
InChI=1S/C33H20/c1-5-25-9-17-29(18-10-25)33(30-19-11-26(6-2)12-20-30,31-21-13-27(7-3)14-22-31)32-23-15-28(8-4)16-24-32/h1-4,9-24H | |
Source | PubChem | |
URL | https://pubchem.ncbi.nlm.nih.gov | |
Description | Data deposited in or computed by PubChem | |
InChI Key |
JGUCYLISNORQFW-UHFFFAOYSA-N | |
Source | PubChem | |
URL | https://pubchem.ncbi.nlm.nih.gov | |
Description | Data deposited in or computed by PubChem | |
Canonical SMILES |
C#CC1=CC=C(C=C1)C(C2=CC=C(C=C2)C#C)(C3=CC=C(C=C3)C#C)C4=CC=C(C=C4)C#C | |
Source | PubChem | |
URL | https://pubchem.ncbi.nlm.nih.gov | |
Description | Data deposited in or computed by PubChem | |
Molecular Formula |
C33H20 | |
Source | PubChem | |
URL | https://pubchem.ncbi.nlm.nih.gov | |
Description | Data deposited in or computed by PubChem | |
Molecular Weight |
416.5 g/mol | |
Source | PubChem | |
URL | https://pubchem.ncbi.nlm.nih.gov | |
Description | Data deposited in or computed by PubChem | |
Retrosynthesis Analysis
AI-Powered Synthesis Planning: Our tool employs the Template_relevance Pistachio, Template_relevance Bkms_metabolic, Template_relevance Pistachio_ringbreaker, Template_relevance Reaxys, Template_relevance Reaxys_biocatalysis model, leveraging a vast database of chemical reactions to predict feasible synthetic routes.
One-Step Synthesis Focus: Specifically designed for one-step synthesis, it provides concise and direct routes for your target compounds, streamlining the synthesis process.
Accurate Predictions: Utilizing the extensive PISTACHIO, BKMS_METABOLIC, PISTACHIO_RINGBREAKER, REAXYS, REAXYS_BIOCATALYSIS database, our tool offers high-accuracy predictions, reflecting the latest in chemical research and data.
Strategy Settings
Precursor scoring | Relevance Heuristic |
---|---|
Min. plausibility | 0.01 |
Model | Template_relevance |
Template Set | Pistachio/Bkms_metabolic/Pistachio_ringbreaker/Reaxys/Reaxys_biocatalysis |
Top-N result to add to graph | 6 |
Feasible Synthetic Routes
Q1: What unique structural features contribute to the formation of porous networks with Tetrakis(4-ethynylphenyl)methane?
A1: this compound possesses a tetrahedral geometry with four ethynyl groups extending outwards. This structure facilitates its participation in Sonogashira coupling reactions with dihalo building blocks, leading to the formation of three-dimensional networks. The rigidity of the tetrahedral core and the spatial arrangement of the ethynyl groups contribute to the formation of microporous structures. [, , , ]
Q2: How can the porosity of networks derived from this compound be tuned?
A2: The porosity of these networks can be tuned through various strategies. One approach involves varying the reaction conditions during the Sonogashira coupling reaction. For instance, gradual addition of the dihalo building block to a mixture containing this compound under Sonogashira coupling conditions has been shown to yield tubular materials, showcasing the influence of reaction kinetics on morphology. [] Additionally, incorporating different linkers or functional groups within the network structure can modify pore size and surface properties. [, ]
Q3: What catalytic applications have been explored with this compound-based materials?
A3: this compound-derived porous organic polymers (POPs) have demonstrated promising catalytic activities. For example, incorporating imidazolium salts into the network through Sonogashira coupling with diiodoimidazolium salts yields materials (T-IM) with catalytic activity in the conversion of carbon dioxide into cyclic carbonates. [] Additionally, hyper-cross-linked polyarylacetylene networks prepared using this compound exhibit high surface areas and can function as heterogeneous acid catalysts in reactions like aldehyde/ketone acetalization and carboxylic acid esterification. []
Q4: What role does weak hydrogen bonding play in the crystal structure of this compound?
A4: Crystallographic studies have shown that this compound molecules assemble into interwoven diamondoid lattices. This arrangement is stabilized in part by weak C−H···C≡C hydrogen bonds between the acetylenic groups, highlighting the significance of these interactions in directing the solid-state structure. [, ]
Q5: How do structural modifications to this compound affect the properties of the resulting polymers?
A5: Introducing specific moieties into the polymer backbone can significantly alter material properties. For instance, incorporating triphenylamine or azobenzene units into this compound-based polymers increases the density of electron-donating nitrogen sites within the porous framework. This modification enhances carbon dioxide adsorption due to favorable interactions between the Lewis acidic carbon dioxide molecules and the basic nitrogen sites. []
Q6: Have computational methods been employed to study this compound and its derivatives?
A6: Yes, computational chemistry has been utilized to investigate the properties and behavior of this compound systems. For instance, the cyclic periodic wave function (CPWF) approach at the AM1 and PM3 semiempirical levels has been used to study infinitely periodic solid-state systems stabilized by C−H···π interactions between this compound units, demonstrating the utility of computational tools in understanding these materials. []
Q7: What analytical techniques are commonly used to characterize this compound-based materials?
A7: Characterization of these materials often involves a combination of techniques. Common methods include thermogravimetric analysis (TGA) to assess thermal stability, infrared (IR) spectroscopy to identify functional groups, scanning electron microscopy (SEM) to visualize morphology, and Brunauer-Emmett-Teller (BET) analysis to determine surface area and porosity. Solid-state 13C NMR spectroscopy can provide insights into the network structure. [, ]
Q8: What makes this compound suitable for creating organic-inorganic hybrid materials?
A8: this compound's ethynyl groups can participate in hydrosilylation reactions with bisdimethylsilyl compounds like 1,1,3,3-tetramethyldisiloxane (TMDS) or 1,4-bis(dimethylsilyl)benzene (BDMSB). This reaction allows for the creation of organic-inorganic hybrid gels with tunable optical properties based on the σ–π conjugation within the network structure. []
Q9: Can this compound be used to create fluorescent materials?
A9: Yes, this compound can be used to prepare fluorescent porous organic polymers. By polymerizing it with fluorescent monomers like diketopyrrolopyrrole derivatives, the resulting polymers inherit the fluorescent nature of the incorporated monomer. These materials can then be further developed for applications such as sensing, taking advantage of changes in fluorescence upon interaction with specific analytes. []
Disclaimer and Information on In-Vitro Research Products
Please be aware that all articles and product information presented on BenchChem are intended solely for informational purposes. The products available for purchase on BenchChem are specifically designed for in-vitro studies, which are conducted outside of living organisms. In-vitro studies, derived from the Latin term "in glass," involve experiments performed in controlled laboratory settings using cells or tissues. It is important to note that these products are not categorized as medicines or drugs, and they have not received approval from the FDA for the prevention, treatment, or cure of any medical condition, ailment, or disease. We must emphasize that any form of bodily introduction of these products into humans or animals is strictly prohibited by law. It is essential to adhere to these guidelines to ensure compliance with legal and ethical standards in research and experimentation.