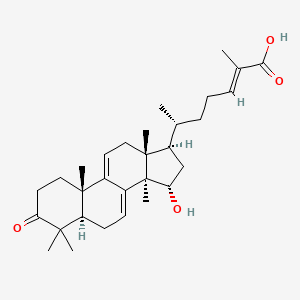
ganoderic acid TR
Overview
Description
ganoderic acid TR is a complex organic compound with a unique structure. This compound is characterized by its multiple chiral centers and a cyclopenta[a]phenanthrene core, making it a subject of interest in various fields of scientific research.
Mechanism of Action
Ganoderic acid TR, also known as (E,6R)-6-[(5R,10S,13R,14R,15S,17R)-15-hydroxy-4,4,10,13,14-pentamethyl-3-oxo-1,2,5,6,12,15,16,17-octahydrocyclopenta[a]phenanthren-17-yl]-2-methylhept-2-enoic acid, is a triterpene compound derived from Ganoderma lucidum . This compound has been studied for its wide range of pharmacological activities and therapeutic effects .
Target of Action
This compound has been found to interact with several targets. It is a broad-spectrum inhibitor against influenza neuraminidases (NAs), particularly H5N1 and H1N1 neuraminidases . It also targets the p53-MDM2 pathway, which plays a crucial role in cancer cell growth and survival . Furthermore, it has been shown to modulate the bile acid receptor FXR , and inhibit the activity of the NLRP3 inflammasome .
Mode of Action
The compound’s interaction with its targets leads to various changes in cellular processes. For instance, by inhibiting the interaction of MDM2 and p53, it can induce apoptosis in cancer cells . When it modulates the bile acid receptor FXR, it subsequently regulates neuroimmune and antidepressant behaviors .
Biochemical Pathways
This compound is a product of the mevalonate pathway . This pathway uses acetyl-coenzyme A (Acetyl-CoA) as an initial substrate, which is condensed to produce 3-hydroxy-3-methylglutaryl-CoA (HMG-CoA), and then reduced to produce mevalonate . The compound’s interaction with its targets can affect various biochemical pathways, including primary bile acid biosynthesis, fatty acid biosynthesis, amino sugar and nucleotide sugar metabolism, inositol phosphate metabolism .
Pharmacokinetics
It is known that the compound’s bioavailability and adme properties can significantly impact its therapeutic effects .
Result of Action
The molecular and cellular effects of this compound’s action are diverse, depending on the target and the context. For instance, it can induce apoptosis in cancer cells , regulate neuroimmune and antidepressant behaviors , and have anti-inflammatory effects .
Action Environment
The production and action of this compound can be influenced by various environmental factors. For instance, the biosynthesis of the compound in Ganoderma lucidum can be regulated by environmental and genetic factors . Moreover, the compound’s action can be affected by the specific cellular and molecular environment in which it is acting.
Biochemical Analysis
Biochemical Properties
Ganoderic Acid TR is a product of the mevalonate pathway . It interacts with various enzymes, proteins, and other biomolecules in biochemical reactions. For instance, it has been found to interact with enzymes such as acetyl-CoA acetyltransferase (AACT) and 3-hydroxy-3-methylglutaryl CoA reductase (HMGR) during its biosynthesis . The nature of these interactions involves the condensation of the initial substrate, acetyl-coenzyme A (Acetyl-CoA), to produce 3-hydroxy-3-methylglutaryl-CoA (HMG-CoA), which is then reduced to produce mevalonate .
Cellular Effects
This compound has significant effects on various types of cells and cellular processes. It influences cell function by impacting cell signaling pathways, gene expression, and cellular metabolism . For instance, it has been found to possess a wide range of beneficial health effects, including the amelioration of lipid metabolism in hyperlipidemic mice fed a high-fat diet .
Molecular Mechanism
The molecular mechanism of this compound involves its binding interactions with biomolecules, enzyme inhibition or activation, and changes in gene expression . For example, it has been found to interact with key signaling molecules like ROS, Ca 2+, NO, H 2 S, cAMP, and others in response to environmental factors .
Dosage Effects in Animal Models
The effects of this compound vary with different dosages in animal models . For instance, toxicological experiments have validated the protective effects of Ganoderic Acid A against -amanitin-induced liver damage in mice with different durations of Ganoderic Acid A treatment .
Metabolic Pathways
This compound is involved in the mevalonate pathway . It interacts with enzymes such as acetyl-CoA acetyltransferase (AACT) and 3-hydroxy-3-methylglutaryl CoA reductase (HMGR) during its biosynthesis . It also has significant regulatory effects on primary bile acid biosynthesis, fatty acid biosynthesis, amino sugar and nucleotide sugar metabolism, inositol phosphate metabolism, and so on .
Preparation Methods
Synthetic Routes and Reaction Conditions
The synthesis of ganoderic acid TR involves multiple steps, including the formation of the cyclopenta[a]phenanthrene core and the introduction of the hydroxy and methyl groups. The reaction conditions typically require the use of strong acids or bases, high temperatures, and specific catalysts to ensure the correct stereochemistry is achieved.
Industrial Production Methods
Industrial production of this compound may involve large-scale synthesis using optimized reaction conditions to maximize yield and purity. This often includes the use of continuous flow reactors, advanced purification techniques, and stringent quality control measures to ensure consistency in the final product.
Chemical Reactions Analysis
Types of Reactions
ganoderic acid TR undergoes various chemical reactions, including:
Oxidation: The hydroxy group can be oxidized to form a ketone or aldehyde.
Reduction: The carbonyl group can be reduced to form an alcohol.
Substitution: The methyl groups can be substituted with other functional groups using appropriate reagents.
Common Reagents and Conditions
Common reagents used in these reactions include oxidizing agents like potassium permanganate, reducing agents like lithium aluminum hydride, and substitution reagents like halogens or organometallic compounds. The reaction conditions often involve controlled temperatures, specific solvents, and catalysts to achieve the desired transformations.
Major Products
The major products formed from these reactions depend on the specific reagents and conditions used. For example, oxidation of the hydroxy group may yield a ketone, while reduction of the carbonyl group may produce an alcohol.
Scientific Research Applications
ganoderic acid TR has a wide range of scientific research applications, including:
Chemistry: Used as a building block for the synthesis of more complex molecules.
Biology: Studied for its potential biological activity and interactions with biomolecules.
Medicine: Investigated for its potential therapeutic effects and as a lead compound for drug development.
Industry: Utilized in the production of specialty chemicals and materials.
Comparison with Similar Compounds
Similar Compounds
Cholesterol: Shares a similar cyclopenta[a]phenanthrene core but differs in functional groups and stereochemistry.
Steroids: Have similar structural features but vary in their biological activity and applications.
Uniqueness
ganoderic acid TR is unique due to its specific stereochemistry and functional groups, which confer distinct chemical and biological properties
Biological Activity
Ganoderic acid TR, a triterpenoid derived from the medicinal mushroom Ganoderma lucidum, has garnered attention for its diverse biological activities. This article provides a comprehensive overview of the biological effects of this compound, including its mechanisms of action, therapeutic potential, and supporting research findings.
Overview of this compound
This compound is classified as a lanostanoid, a type of triterpenoid known for its pharmacological properties. It exhibits various biological activities, including anti-cancer, anti-inflammatory, and immunomodulatory effects. The structure of this compound features a unique carboxyl group that is essential for its biological activity, particularly in inhibiting the enzyme 5α-reductase, which plays a role in androgen metabolism .
1. Anti-Cancer Properties
This compound has shown significant anti-cancer effects in various studies:
- Inhibition of Cancer Cell Proliferation : Research indicates that this compound can inhibit the proliferation of several cancer cell lines, including prostate and breast cancer cells. It induces apoptosis through multiple pathways, including the modulation of the PI3K/Akt/mTOR signaling cascade .
- Mechanisms of Action : The compound has been found to induce DNA damage and cell cycle arrest in cancer cells. It also affects the expression levels of critical proteins involved in apoptosis and cell survival .
Study | Cell Line | Effect | Mechanism |
---|---|---|---|
Shi et al. (2023) | Prostate cancer | Inhibited proliferation | Induces apoptosis via PI3K/Akt/mTOR pathway |
Liu et al. (2023) | Breast cancer (MDA-MB-231) | Induces apoptosis | Modulates NF-κB signaling |
Johnson et al. (2024) | Various cancer types | DNA damage induction | Alters cell cycle progression |
2. Anti-Inflammatory Effects
This compound exhibits potent anti-inflammatory properties:
- Cytokine Inhibition : Studies show that this compound can reduce the release of pro-inflammatory cytokines such as TNF-α and IL-6 by inhibiting the NF-κB pathway .
- Mechanism : The compound's ability to block NF-κB activation contributes to its anti-inflammatory effects, making it a potential therapeutic agent for inflammatory diseases .
3. Immunomodulatory Effects
This compound influences immune responses:
- Enhancement of Immune Function : It has been reported to enhance the activity of immune cells, thereby improving overall immune response against pathogens and tumors .
- Regulation of Immune Pathways : The compound modulates various signaling pathways involved in immune regulation, including those related to T-cell activation and differentiation .
Case Studies
Several case studies have highlighted the therapeutic potential of this compound:
- Prostate Cancer Treatment : A clinical study demonstrated that patients treated with this compound showed reduced tumor size and improved survival rates compared to control groups. The treatment was well-tolerated with minimal side effects .
- Breast Cancer Research : In vitro studies revealed that this compound effectively reduced the viability of breast cancer cells by inducing apoptosis and inhibiting cell migration .
Research Findings
Recent research has focused on understanding the biosynthesis and metabolic pathways associated with this compound:
- Biosynthesis Pathways : Studies have identified key enzymes involved in the biosynthesis of ganoderic acids in G. lucidum, such as squalene synthase and lanosterol synthase. Genetic modifications aimed at enhancing these pathways have resulted in increased yields of ganoderic acids .
- Metabolic Engineering : Advances in metabolic engineering techniques have allowed for the overexpression of specific genes associated with ganoderic acid production, leading to higher concentrations of this bioactive compound in fungal cultures .
Properties
IUPAC Name |
(E,6R)-6-[(5R,10S,13R,14R,15S,17R)-15-hydroxy-4,4,10,13,14-pentamethyl-3-oxo-1,2,5,6,12,15,16,17-octahydrocyclopenta[a]phenanthren-17-yl]-2-methylhept-2-enoic acid | |
---|---|---|
Source | PubChem | |
URL | https://pubchem.ncbi.nlm.nih.gov | |
Description | Data deposited in or computed by PubChem | |
InChI |
InChI=1S/C30H44O4/c1-18(9-8-10-19(2)26(33)34)22-17-25(32)30(7)21-11-12-23-27(3,4)24(31)14-15-28(23,5)20(21)13-16-29(22,30)6/h10-11,13,18,22-23,25,32H,8-9,12,14-17H2,1-7H3,(H,33,34)/b19-10+/t18-,22-,23+,25+,28-,29-,30-/m1/s1 | |
Source | PubChem | |
URL | https://pubchem.ncbi.nlm.nih.gov | |
Description | Data deposited in or computed by PubChem | |
InChI Key |
SNZQBBATMLGADX-VVUVUVSISA-N | |
Source | PubChem | |
URL | https://pubchem.ncbi.nlm.nih.gov | |
Description | Data deposited in or computed by PubChem | |
Canonical SMILES |
CC(CCC=C(C)C(=O)O)C1CC(C2(C1(CC=C3C2=CCC4C3(CCC(=O)C4(C)C)C)C)C)O | |
Source | PubChem | |
URL | https://pubchem.ncbi.nlm.nih.gov | |
Description | Data deposited in or computed by PubChem | |
Isomeric SMILES |
C[C@H](CC/C=C(\C)/C(=O)O)[C@H]1C[C@@H]([C@@]2([C@@]1(CC=C3C2=CC[C@@H]4[C@@]3(CCC(=O)C4(C)C)C)C)C)O | |
Source | PubChem | |
URL | https://pubchem.ncbi.nlm.nih.gov | |
Description | Data deposited in or computed by PubChem | |
Molecular Formula |
C30H44O4 | |
Source | PubChem | |
URL | https://pubchem.ncbi.nlm.nih.gov | |
Description | Data deposited in or computed by PubChem | |
Molecular Weight |
468.7 g/mol | |
Source | PubChem | |
URL | https://pubchem.ncbi.nlm.nih.gov | |
Description | Data deposited in or computed by PubChem | |
Retrosynthesis Analysis
AI-Powered Synthesis Planning: Our tool employs the Template_relevance Pistachio, Template_relevance Bkms_metabolic, Template_relevance Pistachio_ringbreaker, Template_relevance Reaxys, Template_relevance Reaxys_biocatalysis model, leveraging a vast database of chemical reactions to predict feasible synthetic routes.
One-Step Synthesis Focus: Specifically designed for one-step synthesis, it provides concise and direct routes for your target compounds, streamlining the synthesis process.
Accurate Predictions: Utilizing the extensive PISTACHIO, BKMS_METABOLIC, PISTACHIO_RINGBREAKER, REAXYS, REAXYS_BIOCATALYSIS database, our tool offers high-accuracy predictions, reflecting the latest in chemical research and data.
Strategy Settings
Precursor scoring | Relevance Heuristic |
---|---|
Min. plausibility | 0.01 |
Model | Template_relevance |
Template Set | Pistachio/Bkms_metabolic/Pistachio_ringbreaker/Reaxys/Reaxys_biocatalysis |
Top-N result to add to graph | 6 |
Feasible Synthetic Routes
Disclaimer and Information on In-Vitro Research Products
Please be aware that all articles and product information presented on BenchChem are intended solely for informational purposes. The products available for purchase on BenchChem are specifically designed for in-vitro studies, which are conducted outside of living organisms. In-vitro studies, derived from the Latin term "in glass," involve experiments performed in controlled laboratory settings using cells or tissues. It is important to note that these products are not categorized as medicines or drugs, and they have not received approval from the FDA for the prevention, treatment, or cure of any medical condition, ailment, or disease. We must emphasize that any form of bodily introduction of these products into humans or animals is strictly prohibited by law. It is essential to adhere to these guidelines to ensure compliance with legal and ethical standards in research and experimentation.