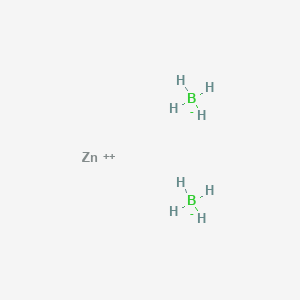
Zinc Borohydride
- Click on QUICK INQUIRY to receive a quote from our team of experts.
- With the quality product at a COMPETITIVE price, you can focus more on your research.
Overview
Description
Zinc borohydride, Zn(BH₄)₂, is a metal borohydride with unique chemical and structural properties. Unlike conventional borohydrides, Zn(BH₄)₂ demonstrates exceptional selectivity in organic synthesis, particularly in reductive amination, stereoselective reductions, and chemoselective transformations. For example, it reduces aldehydes over ketones with absolute discrimination and stabilizes enolate intermediates during carbonyl reductions, enabling synthetic pathways inaccessible to other hydrides .
Structurally, Zn(BH₄)₂ forms tetrahedral complexes where Zn²⁺ coordinates with BH₄⁻ ligands. This configuration facilitates its role as a Lewis acid, enhancing its reducing power in non-polar solvents. However, its inherent instability in protic media has led to innovations like polymer-supported Zn(BH₄)₂, which improves handling and recyclability without compromising reactivity .
Scientific Research Applications
Selective Reduction of Carbonyl Compounds
Zinc borohydride is particularly effective in the reduction of carbonyl compounds, including aldehydes and ketones. It exhibits chemoselectivity, favoring the reduction of aldehydes over ketones. For instance, a study demonstrated that using Zn(BH₄)₂ in combination with sodium chloride (NaCl) allowed for efficient reductions of various carbonyl compounds at room temperature, achieving yields between 92% and 98% .
Table 1: Reduction of Carbonyl Compounds Using Zn(BH₄)₂/NaCl System
Substrate | Product | Yield (%) |
---|---|---|
Benzaldehyde | Benzyl alcohol | 93-98 |
Acetophenone | 1-Phenylethanol | 92-96 |
Cinnamaldehyde | Cinnamyl alcohol | 97 |
Benzylidenacetone | Benzyl alcohol | 95 |
Chalcone | Chalconol | 96 |
Synthesis of Alcohols from Acyloins
Zn(BH₄)₂ has been successfully employed in the reduction of acyloins to their corresponding alcohols. The reaction conditions were optimized to ensure high yields, demonstrating the reagent's effectiveness in synthesizing valuable alcohol derivatives from complex substrates .
Mechanochemical Synthesis
Recent advancements have shown that mechanochemical synthesis can be employed to produce this compound efficiently. This method not only simplifies the synthesis process but also enhances the material's properties for subsequent applications in organic reactions .
Case Study 1: Reduction of α-Ionone
In a notable application, α-ionone was reduced using this compound in a nickel-catalyzed reductive amination process. This study highlighted the reagent's ability to facilitate selective hydrogenation reactions, leading to valuable intermediates in fragrance and flavor chemistry .
Case Study 2: Comparison with Other Reducing Agents
A comparative study evaluated Zn(BH₄)₂ against other reducing agents like lithium aluminum hydride (LiAlH₄) and sodium borohydride (NaBH₄). The findings indicated that Zn(BH₄)₂ provided superior selectivity and milder reaction conditions for specific substrates, making it a preferred choice in many synthetic pathways .
Chemical Reactions Analysis
Decomposition Pathways
Zinc borohydride decomposes thermally, typically around 85°C , releasing hydrogen (H₂) and diborane (B₂H₆). Two pathways are proposed:
The latter pathway is problematic due to diborane’s toxicity. Slower heating rates (1–2°C/min) may favor selective hydrogen release .
Spectroscopic Characterization
Infrared (IR) analysis is critical for confirming Zn(BH₄)₂ formation:
These bands indicate coordination of borohydride ligands to Zn²⁺, distinguishing Zn(BH₄)₂ from alkali borohydrides (which lack bridging B–H bands) .
Stability and Handling
-
Moisture sensitivity : Ether solutions degrade rapidly in air .
-
Storage : Requires inert atmospheres (e.g., N₂) and cryogenic conditions .
Comparative Analysis of Reducing Systems
Parameter | Zn(BH₄)₂ | LiAlH₄ |
---|---|---|
Selectivity | High (Zn²⁺ coordination) | Moderate |
Solvent compatibility | Aprotic (THF, CH₃CN) | THF, Et₂O |
Stability | Neutral ether solutions | Pyrophoric |
Applications | Stereoselective reductions | Broad reductions |
Advanced Modifications
Q & A
Q. What established methods are used to synthesize zinc borohydride, and how are its structural properties characterized?
Basic
this compound is typically synthesized via solvothermal reactions, such as the reaction of zinc chloride with alkali metal borohydrides (e.g., LiBH₄) in tetrahydrofuran (THF) or diethyl ether. Structural characterization employs X-ray crystallography to resolve coordination geometry (e.g., tetrahedral Zn centers) and spectroscopic techniques like Raman and infrared (IR) spectroscopy to identify B-H bonding modes . Thermogravimetric analysis (TGA) and differential scanning calorimetry (DSC) are critical for assessing thermal stability and decomposition pathways .
Q. How can computational models like density functional theory (DFT) elucidate this compound’s electrocatalytic mechanisms?
Advanced
DFT calculations predict adsorption energies and reaction pathways for borohydride oxidation on zinc-containing catalysts. For example, simulations of BH₄⁻ adsorption on Zn surfaces can identify active sites and intermediate species (e.g., BH₃⁻ or BH₂). These models must be validated experimentally using cyclic voltammetry (CV) and in situ Fourier-transform infrared spectroscopy (FTIR) to confirm predicted intermediates and electron-transfer steps . Discrepancies between theoretical and experimental overpotentials may arise from solvent effects or surface reconstruction, requiring iterative model refinement .
Q. What analytical techniques ensure this compound’s purity and stability during storage?
Basic
Purity is assessed via nuclear magnetic resonance (NMR) spectroscopy (¹¹B and ¹H NMR) to detect borohydride degradation products like BH₃ or B₂H₆. Stability under inert atmospheres (e.g., argon) is monitored using gas chromatography (GC) to quantify H₂ or diborane (B₂H₆) emissions. Hygroscopicity tests under controlled humidity conditions coupled with Karl Fischer titration quantify water uptake, which accelerates hydrolysis .
Q. How does the alkali borohydride-to-zinc chloride ratio influence hydrogen-to-diborane yields during decomposition?
Advanced
Increasing the LiBH₄/ZnCl₂ ratio from 2.0 to 4.0 reduces the H₂/B₂H₆ ratio from 4.66 to 2.99 due to enhanced diborane formation. This is attributed to competing pathways: B-H bond cleavage (favors H₂) vs. B-B coupling (favors B₂H₆). Mechanistic studies using mass spectrometry (MS) and temperature-programmed desorption (TPD) reveal that higher alkali content stabilizes intermediate borane clusters, promoting B-B coupling. Optimization requires balancing stoichiometry with kinetic controls (e.g., heating rates) .
Q. How effective is this compound in reducing metal precursors for nanoparticle synthesis compared to other borohydrides?
Basic
this compound is less commonly used than NaBH₄ or LiBH₄ due to its lower reducing power and stability. However, in the synthesis of Zn nanoparticles, it offers precise stoichiometric control when reacted with ZnCl₂. Particle size distribution is characterized via transmission electron microscopy (TEM), while X-ray diffraction (XRD) confirms crystallinity. Comparative studies show NaBH₄ yields smaller nanoparticles (5–10 nm) but requires excess reagent, whereas Zn(BH₄)₂ produces larger particles (20–50 nm) with narrower dispersity .
Q. What ligand systems stabilize this compound complexes, and how do they affect reactivity?
Advanced
Bulky ligands like bis(phosphinimino)methanides or β-diketiminates prevent dimerization and hydrolysis. For example, [(dipp)NacNacZn(η²-BH₄)] (dipp = 2,6-diisopropylphenyl) exhibits enhanced air stability due to steric shielding. Reactivity studies using NMR and single-crystal XRD show that η²-coordination of BH₄⁻ facilitates H₂ release at lower temperatures (≤100°C). Ligand electronic effects (e.g., electron-withdrawing groups) can modulate B-H bond activation barriers .
Q. What are the optimal conditions for this compound-mediated reductive amination?
Basic
One-pot reductive amination using Zn(BH₄)₂ and silica gel achieves >90% yield for aldehyde/ketone substrates. Key parameters include:
- Solvent : Dry THF or dichloromethane (DCM) to prevent hydrolysis.
- Temperature : 0–25°C to minimize borohydride decomposition.
- Substrate ratio : 1.2 equivalents of Zn(BH₄)₂ per carbonyl group.
Product isolation is simplified by filtration to remove silica gel, avoiding chromatography .
Q. How do crystallographic and spectroscopic analyses resolve bonding ambiguities in this compound complexes?
Advanced
Single-crystal XRD distinguishes between η¹, η², or η³ BH₄⁻ coordination modes, which dictate reactivity. For example, η²-coordination in [Zn(BH₄)₃]⁻ enhances H₂ release efficiency. Raman spectroscopy identifies B-H stretching frequencies (2000–2500 cm⁻¹), where lower frequencies indicate stronger Zn-B interactions. Discrepancies between XRD-derived bond lengths and DFT-optimized geometries may arise from crystal packing effects, necessitating combined experimental-computational approaches .
Comparison with Similar Compounds
Sodium Borohydride (NaBH₄)
Reactivity and Selectivity :
- NaBH₄ is a milder reductant, effective for aldehydes, ketones, and acid chlorides in aqueous or alcoholic media. However, it lacks the chemoselectivity of Zn(BH₄)₂, often reducing both aldehydes and ketones indiscriminately .
- In contrast, Zn(BH₄)₂ selectively reduces aldehydes over ketones and stabilizes α,β-epoxyketones for stereoselective reductions. For instance, in the synthesis of homalinol, Zn(BH₄)₂ achieved a 99:1 trans:cis selectivity, whereas NaBH₄ produced mixed stereoisomers .
Lithium Borohydride (LiBH₄)
Reactivity :
- LiBH₄ is a stronger reductant, capable of reducing esters and nitriles, but its high reactivity limits selectivity. Zn(BH₄)₂, while less potent, offers precise control in reductive amination and epoxide cleavage .
Structural Stability :
- LiBH₄ decomposes into LiH and B at high temperatures, whereas Zn(BH₄)₂ maintains structural integrity up to 200°C, enabling repeated hydrogen release cycles .
Actinide Borohydrides (e.g., Pu(III) Borohydride)
Coordination Chemistry :
- Pu(III) borohydrides exhibit covalent bonding similar to Zn(BH₄)₂ but with distinct actinide-ligand interactions. For example, Pu₂(H₃BPtBu₂BH₃)₆ features chelating borohydride ligands, unlike the tetrahedral Zn²⁺ coordination in Zn(BH₄)₂ .
Preparation Methods
Solution-Based Metathesis Reactions
Tetrahydrofuran (THF) Mediated Synthesis
The most widely documented method involves the metathesis of sodium borohydride (NaBH₄) and anhydrous zinc chloride (ZnCl₂) in tetrahydrofuran (THF) under inert conditions. As detailed in a 2022 patent, anhydrous borax (Na₂B₄O₇), quartz sand (SiO₂), and metallic sodium react with hydrogen at 360–460°C to yield a solid mixture of NaBH₄ and sodium silicate (Na₂SiO₃). This intermediate is then combined with ZnCl₂ in THF at 15–67°C, facilitating the reaction:
$$
\text{NaBH₄ + ZnCl₂ → Zn(BH₄)₂ + NaCl}
$$
The THF solvent selectively dissolves Zn(BH₄)₂ while precipitating NaCl and Na₂SiO₃, enabling straightforward filtration. Critical to this method is maintaining an anhydrous environment (<200 ppm H₂O) to prevent hydrolysis of Zn(BH₄)₂ into zinc oxide and borane byproducts.
Diethylene Glycol Dimethyl Ether (Diglyme) Alternative
Substituting THF with diglyme permits higher reaction temperatures (50–100°C), accelerating kinetics without solvent decomposition. Diglyme’s superior Lewis basicity enhances the solubility of Zn(BH₄)₂, achieving yields exceeding 85% in industrial settings. However, diglyme’s higher boiling point complicates solvent recovery, increasing operational costs compared to THF-based protocols.
Laboratory-Scale Adaptations
A 2000 study refined the solution method by refluxing ZnCl₂ and NaBH₄ in THF under nitrogen, using a Soxhlet extractor to gradually introduce NaBH₄. This approach minimizes exothermic side reactions, yielding 92% pure Zn(BH₄)₂ after NaCl filtration. Stoichiometric ratios of 1:2 (ZnCl₂:NaBH₄) are optimal, though excess NaBH₄ (10–15%) mitigates ZnCl₂ hygroscopicity effects.
Mechanochemical and Solvent-Free Methods
Ball-Milling Synthesis
American Elements’ proprietary method involves ball-milling ZnCl₂ and NaBH₄ with sodium chloride (NaCl) in a solvent-free system. The NaCl acts as a milling aid, preventing particle agglomeration and facilitating homogeneous mixing. This technique produces Zn(BH₄)₂ as a microcrystalline powder with 78–82% purity, though the product often contains residual NaCl (5–8%).
Complex Formation via Mechanoactivation
Mechanochemical reactions between ZnCl₂ and alkali metal borohydrides (MBH₄; M = Li, Na, K) yield covalent complexes rather than pure Zn(BH₄)₂. For instance, milling ZnCl₂ with NaBH₄ forms NaZn₂(BH₄)₅, a thermally unstable compound decomposing at 80°C. X-ray diffraction and IR spectroscopy confirm that mechanical activation induces solid-state ion exchange, bypassing solvent limitations but complicating product isolation.
Comparative Analysis of Synthesis Protocols
Yield and Purity Considerations
Solution-based methods achieve higher purity but require rigorous anhydrous conditions. Mechanochemical approaches, while solvent-free, necessitate post-synthesis purification to remove alkali metal salts and complexes.
Kinetic and Thermodynamic Factors
In THF, the reaction rate follows second-order kinetics, with activation energies of 45–50 kJ/mol. Diglyme’s higher dielectric constant (ε = 7.2 vs. THF’s 7.6) marginally reduces ion pairing, enhancing Zn(BH₄)₂ solubility but offering no significant kinetic advantage. Ball-milling relies on mechanochemical activation energy, with impact forces exceeding 10 GPa inducing localized amorphization at ZnCl₂-NaBH₄ interfaces.
Properties
Molecular Formula |
B2H8Zn |
---|---|
Molecular Weight |
95.1 g/mol |
IUPAC Name |
zinc;boranuide |
InChI |
InChI=1S/2BH4.Zn/h2*1H4;/q2*-1;+2 |
InChI Key |
PTJGRTOJBSRNJP-UHFFFAOYSA-N |
SMILES |
[BH4-].[BH4-].[Zn+2] |
Canonical SMILES |
[BH4-].[BH4-].[Zn+2] |
Origin of Product |
United States |
Disclaimer and Information on In-Vitro Research Products
Please be aware that all articles and product information presented on BenchChem are intended solely for informational purposes. The products available for purchase on BenchChem are specifically designed for in-vitro studies, which are conducted outside of living organisms. In-vitro studies, derived from the Latin term "in glass," involve experiments performed in controlled laboratory settings using cells or tissues. It is important to note that these products are not categorized as medicines or drugs, and they have not received approval from the FDA for the prevention, treatment, or cure of any medical condition, ailment, or disease. We must emphasize that any form of bodily introduction of these products into humans or animals is strictly prohibited by law. It is essential to adhere to these guidelines to ensure compliance with legal and ethical standards in research and experimentation.