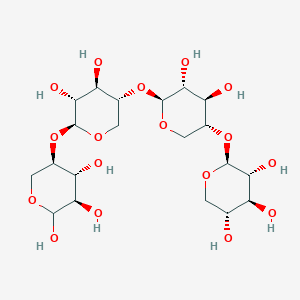
Xylotetraose
- Click on QUICK INQUIRY to receive a quote from our team of experts.
- With the quality product at a COMPETITIVE price, you can focus more on your research.
Description
Xylotetraose is a tetrasaccharide comprised of four D-xylose residues connected by beta(1->4) linkages.
Scientific Research Applications
Gut Microbiota and Sugar Mimics
Xylotetraose is utilized by the ABC transporter of gut microbiota Bifidobacteria. Zhang and Li (2021) explored the potential of this compound as a sugar mimic in the solute-binding protein BlAXBP of the ABC transporter from Bifidobacterium animalis subsp. Lactis (Zhang & Li, 2021).
Enzymatic Hydrolysis and Oligosaccharide Production
Kubata et al. (1995) discovered an exoxylanase from Aeromonas caviae ME-1 that produces this compound from oat spelt xylan, indicating its potential in oligosaccharide production (Kubata et al., 1995). Li et al. (2019) also researched the co-production of functional xylo-oligosaccharides and fermentable sugars from sugarcane bagasse, highlighting this compound's role in this process (Li et al., 2019).
Molecular Dynamics and Free Energy Analysis
The binding of this compound to enzymes like endo-1,4-beta-xylanase II from Trichoderma reesei has been studied using molecular dynamics simulations and free energy analyses, providing insights into enzyme-substrate interactions (Laitinen, Rouvinen, & Peräkylä, 2003).
Food Industry Applications
This compound has been explored in the food industry, like in the study of Zhu et al. (2010), who investigated its effects on the fermentation properties of dough and breadmaking (Zhu, Wang, Huang, Zheng, & Rayas-Duarte, 2010).
Fermentation and Biofuel Production
In the context of biofuel production, studies have focused on the fermentation of xylose, with this compound playing a role in understanding and optimizing this process. For example, Zhou et al. (2012) examined Saccharomyces cerevisiae's ability to ferment xylose for ethanol production (Zhou, Cheng, Wang, Fink, & Stephanopoulos, 2012).
Prebiotics and Nutraceuticals
This compound's potential as a prebiotic has been highlighted in several studies, such as Reddy and Krishnan (2016), who examined its use in food industries due to its prebiotic function (Reddy & Krishnan, 2016). Nieto-Domínguez et al. (2017) also evaluated its prebiotic value based on variations in microorganisms' composition and organic acids profile in fermentations (Nieto-Domínguez et al., 2017).
Environmental Sustainability
Research such as that by Zhang et al. (2020) on seawater hydrothermal pretreatment for producing xylooligosaccharides and fermentable sugars from sugarcane bagasse underlines the environmental sustainability of using this compound (Zhang, Zhang, Lei, Yang, & Jiang, 2020).
Biotechnological Innovations
The role of this compound in biotechnological innovations is evident in studies like Precup et al. (2022), who investigated its production from wheat straw for potential food applications (Precup et al., 2022).
Xylan Biosynthesis
Lee et al. (2012) provided biochemical evidence of this compound's involvement in xylan biosynthesis in Arabidopsis thaliana, contributing to our understanding of plant biomass production (Lee, Zhong, & Ye, 2012).
properties
Molecular Formula |
C20H34O17 |
---|---|
Molecular Weight |
546.5 g/mol |
IUPAC Name |
(2S,3R,4S,5R)-2-[(3R,4R,5R,6S)-6-[(3R,4R,5R,6S)-4,5-dihydroxy-6-[(3R,4R,5R)-4,5,6-trihydroxyoxan-3-yl]oxyoxan-3-yl]oxy-4,5-dihydroxyoxan-3-yl]oxyoxane-3,4,5-triol |
InChI |
InChI=1S/C20H34O17/c21-5-1-32-18(14(27)9(5)22)36-7-3-34-20(16(29)11(7)24)37-8-4-33-19(15(28)12(8)25)35-6-2-31-17(30)13(26)10(6)23/h5-30H,1-4H2/t5-,6-,7-,8-,9+,10+,11+,12+,13-,14-,15-,16-,17?,18+,19+,20+/m1/s1 |
InChI Key |
KPTPSLHFVHXOBZ-BIKCPUHGSA-N |
Isomeric SMILES |
C1[C@H]([C@@H]([C@H]([C@@H](O1)O[C@@H]2CO[C@H]([C@@H]([C@H]2O)O)O[C@@H]3CO[C@H]([C@@H]([C@H]3O)O)O[C@@H]4COC([C@@H]([C@H]4O)O)O)O)O)O |
SMILES |
C1C(C(C(C(O1)OC2COC(C(C2O)O)OC3COC(C(C3O)O)OC4COC(C(C4O)O)O)O)O)O |
Canonical SMILES |
C1C(C(C(C(O1)OC2COC(C(C2O)O)OC3COC(C(C3O)O)OC4COC(C(C4O)O)O)O)O)O |
Origin of Product |
United States |
Disclaimer and Information on In-Vitro Research Products
Please be aware that all articles and product information presented on BenchChem are intended solely for informational purposes. The products available for purchase on BenchChem are specifically designed for in-vitro studies, which are conducted outside of living organisms. In-vitro studies, derived from the Latin term "in glass," involve experiments performed in controlled laboratory settings using cells or tissues. It is important to note that these products are not categorized as medicines or drugs, and they have not received approval from the FDA for the prevention, treatment, or cure of any medical condition, ailment, or disease. We must emphasize that any form of bodily introduction of these products into humans or animals is strictly prohibited by law. It is essential to adhere to these guidelines to ensure compliance with legal and ethical standards in research and experimentation.