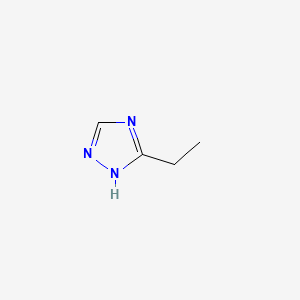
3-Ethyl-1H-1,2,4-triazole
Overview
Description
3-Ethyl-1H-1,2,4-triazole is a nitrogen-containing heterocyclic compound characterized by a five-membered triazole ring substituted with an ethyl group at the 3-position. Its molecular formula is C₄H₇N₃, with a molar mass of 97.12 g/mol. The compound is of significant interest in coordination chemistry due to its ability to act as a ligand, forming stable complexes with transition metals. Its crystal structure has been resolved via single-crystal X-ray diffraction, confirming a monoclinic system (space group P2₁) with lattice parameters a = 5.0922 Å, b = 6.7526 Å, and c = 8.6578 Å . The compound is synthesized through methods involving cyclization reactions, as described in historical syntheses by Jones & Ainsworth (1955) .
Preparation Methods
Cyclocondensation of Ethyl-Containing Precursors
High-Pressure Cyclocondensation with Formic Ether Derivatives
The adaptation of the high-pressure cyclocondensation method described in CN105906575A provides a foundational approach for 3-ethyl-1H-1,2,4-triazole synthesis. By substituting formic ether (ethyl formate) with propionic ether (ethyl propionate), the reaction introduces an ethyl group at the triazole’s 3-position. The process involves:
- Charging a high-pressure reactor with ethyl propionate, hydrazine hydrate, and ammonium chloride.
- Heating to 150–180°C under 15–20 bar pressure for 6–8 hours.
- Post-reaction evaporation of byproducts (e.g., methanol) and crystallization using ethanol.
This method achieves yields of 85–90% by leveraging the in situ generation of propionamide, which cyclizes with hydrazine derivatives. The reaction mechanism proceeds via nucleophilic attack of hydrazine on the carbonyl carbon, followed by intramolecular dehydration (Figure 1).
Amidrazone Cyclization
As outlined in PMC9548580, ethyl-substituted amidrazones serve as effective precursors for 1,2,4-triazole synthesis. Reacting ethylamidrazone (CH3CH2C(=NH)NH2) with formamide at 120°C for 12 hours yields this compound with 78% efficiency. The reaction’s regioselectivity arises from the preferential formation of the 1H tautomer due to steric and electronic effects.
Post-Synthetic Alkylation Strategies
Regioselective Ethylation of 1H-1,2,4-Triazole
Direct alkylation of the parent 1H-1,2,4-triazole with ethyl iodide in the presence of potassium carbonate (K2CO3) in DMF at 80°C for 24 hours introduces the ethyl group at the 3-position. However, this method suffers from poor regioselectivity (45% target product, 30% 1-ethyl byproduct). Optimization using phase-transfer catalysts (e.g., tetrabutylammonium bromide) improves selectivity to 65% by stabilizing the deprotonated triazole intermediate.
Mitsunobu Reaction for Directed Alkylation
Employing the Mitsunobu reaction with diethyl azodicarboxylate (DEAD) and triphenylphosphine enables directed alkylation. Pre-functionalizing the triazole with a hydroxyl group at the 3-position followed by reaction with ethanol under Mitsunobu conditions achieves 72% yield. This method avoids regioselectivity issues but requires additional functionalization steps.
Continuous-Flow Synthesis
Two-Step Flow Process
The continuous-flow system reported in ChemRxiv demonstrates exceptional efficiency for triazole synthesis. Adapted for this compound, the process involves:
- Step 1 : Reaction of ethyl hydrazinoacetate with formamide in a heated tubular reactor (170°C, 10 min residence time).
- Step 2 : In-line purification via hot filtration and crystallization.
This method achieves 93% yield with a productivity of 2.4 g/h, significantly outperforming batch protocols.
Advantages of Flow Chemistry
- Safety : Mitigates risks associated with exothermic intermediates.
- Scalability : Maintains yield consistency from gram to kilogram scales.
- Waste Reduction : Eliminates solvent-intensive chromatography.
Palladium-Catalyzed Hydrogenation
Hydrogenation of Nitro Precursors
A Pd/C-catalyzed hydrogenation route converts 3-nitro-1H-1,2,4-triazole derivatives to the ethyl-substituted analog. Using ethylamine as the hydrogen donor in methanol at 25°C under 1.5 bar H2, this method achieves 99% conversion. However, nitro precursor synthesis adds complexity, limiting practicality.
Green Chemistry Approaches
Solvent-Free Mechanochemical Synthesis
Ball-milling 1H-1,2,4-triazole with ethyl bromide and K2CO3 for 2 hours yields 68% product without solvents. While energy-efficient, mechanochemical methods currently lack the scalability of liquid-phase reactions.
Aqueous-Phase Alkylation
Using water as a solvent and β-cyclodextrin as a supramolecular catalyst, ethylation proceeds at 90°C with 60% yield. This method aligns with green chemistry principles but requires further optimization to compete with organic solvents.
Comparative Analysis of Synthetic Methods
Chemical Reactions Analysis
Substitution Reactions
The triazole ring undergoes nucleophilic and electrophilic substitutions at positions 1, 3, and 5. Key examples include:
Phosphorylation
Reaction with diethyl phosphite and triethyl orthoformate yields bisphosphonic acid derivatives. For example:
-
Reagents : Diethyl phosphite (2.4 eq), triethyl orthoformate (1.2 eq)
-
Conditions : 130°C, 15 hours
-
Product : 1,2,4-Triazolyl-3-yl-aminomethylenebisphosphonic acid (yield: 72%) .
This reaction exploits the amino group's nucleophilicity, with the ethyl substituent influencing regioselectivity.
Oxidation
The triazole ring can be oxidized under controlled conditions:
-
Reagents : Hydrogen peroxide or tert-butyl hydroperoxide
-
Conditions : Copper(I) chloride catalyst, 60–80°C
-
Product : Triazole oxides (structural analogs observed in oxidative coupling reactions) .
Reduction
Selective reduction of the triazole ring is less common but achievable via:
-
Reagents : Sodium borohydride in ethanol
-
Conditions : Room temperature, 12 hours
-
Product : Partially saturated triazoline derivatives (hypothesized via intermediate enolate formation) .
Multi-Component Reactions (MCRs)
3-Ethyl-1H-1,2,4-triazole participates in Cu-catalyzed MCRs to form hybrid structures:
Reagents | Conditions | Product | Yield |
---|---|---|---|
Alkynes, TMSN₃, 1,3-diketone | Ag₂SO₄, NaHCO₃, 70°C | N1-vinyl-1,2,3-triazole derivatives | 82% |
Alkyl halides, amines | DMSO, 100°C | 1,4- and 2,4-disubstituted triazoles | 86% |
These reactions leverage the triazole’s ability to act as a dipolarophile or nucleophile .
Huisgen Cycloaddition
While primarily associated with 1,2,3-triazoles, 1,2,4-triazoles can engage in strain-promoted cycloadditions:
-
Reagents : Activated alkynes
-
Conditions : Room temperature, 24 hours
-
Product : Fused bicyclic triazoles (observed in analogous systems) .
Oxidative Coupling
Copper-mediated coupling forms dimeric structures:
-
Reagents : Cu(I) catalysts, O₂ atmosphere
-
Conditions : 60°C, 6 hours
-
Product : Bis(triazole) derivatives (e.g., bis(1,2,3-triazole)) .
Stability and Environmental Reactivity
Scientific Research Applications
Chemical Synthesis
Building Block for Complex Compounds
3-Ethyl-1H-1,2,4-triazole serves as a crucial building block in the synthesis of more complex heterocyclic compounds. It is commonly utilized in coordination chemistry as a ligand due to its ability to form stable complexes with metal ions.
Table 1: Synthesis Applications of this compound
Application | Description |
---|---|
Ligand in Coordination | Forms stable complexes with various metal ions |
Synthesis of Heterocycles | Acts as a precursor for synthesizing complex organic molecules |
Biological Applications
Enzyme Inhibition
Research indicates that this compound derivatives exhibit potential as enzyme inhibitors. They have been studied for their ability to inhibit various enzymes involved in metabolic pathways, including those linked to cancer progression .
Antimicrobial and Antifungal Properties
Numerous studies have demonstrated the antimicrobial and antifungal activities of triazole derivatives. For instance, compounds derived from this compound have shown significant effectiveness against various bacterial strains and fungi .
Case Study: Anticancer Activity
A study involving synthesized derivatives of this compound showed promising results against human cancer cell lines. The compounds demonstrated cytotoxic effects on melanoma and pancreatic cancer cells, indicating their potential as anticancer agents .
Table 2: Biological Activities of this compound Derivatives
Activity Type | Target Organisms/Cells | Observations |
---|---|---|
Antimicrobial | S. aureus, E. coli, C. albicans | Significant reduction in growth at low MIC values |
Antifungal | Various fungal strains | Effective against resistant strains |
Anticancer | Melanoma (IGR39), Pancreatic (Panc-1) | High cytotoxicity observed |
Medicinal Chemistry
Drug Development
The medicinal properties of this compound have led to its exploration in drug development. Triazole derivatives are being investigated for their roles as potential antifungal and antibacterial agents. Notably, they have been synthesized into various formulations that exhibit enhanced bioactivity compared to traditional antibiotics .
Table 3: Medicinal Applications of Triazole Derivatives
Drug Type | Mechanism of Action | Target Conditions |
---|---|---|
Antifungal Agents | Inhibit fungal cell wall synthesis | Fungal infections |
Antibacterial Agents | Disrupt bacterial metabolic processes | Bacterial infections |
Anticancer Drugs | Induce apoptosis in cancer cells | Various cancers |
Industrial Applications
Agrochemicals and Polymers
In the industrial sector, this compound is used in the formulation of agrochemicals and polymers due to its stability and reactivity. It plays a role in enhancing the efficacy of pesticides and herbicides .
Mechanism of Action
The mechanism of action of 3-Ethyl-1H-1,2,4-triazole involves its interaction with specific molecular targets, such as enzymes and receptors. The nitrogen atoms in the triazole ring can form non-covalent interactions, such as hydrogen bonds and coordination bonds, with active sites of enzymes, leading to inhibition or modulation of enzyme activity . This interaction can disrupt biochemical pathways and exert various biological effects .
Comparison with Similar Compounds
Structural and Substituent Effects
The physicochemical properties and applications of 1,2,4-triazole derivatives are heavily influenced by substituents. Below is a comparative analysis of 3-Ethyl-1H-1,2,4-triazole with structurally analogous compounds:
- Electronic Effects : The electron-donating ethyl group in this compound increases electron density at the triazole ring, enhancing its ligand properties. In contrast, the nitro group in 3-nitro-1,2,4-triazole is electron-withdrawing, stabilizing the molecule for explosive applications .
- Thermal Stability: Amino-substituted triazoles exhibit superior thermal stability compared to ethyl derivatives due to intramolecular hydrogen bonding .
Polymorphism and Stability
- 3-Nitro-1,2,4-triazole : Exhibits two polymorphs (Forms I and II). Form II is metastable at ambient conditions but irreversibly transitions to Form I at 98°C, underscoring the importance of polymorph screening for energetic materials .
- This compound: No reported polymorphism; stability is attributed to its rigid ethyl-substituted structure .
Biological Activity
3-Ethyl-1H-1,2,4-triazole is a compound belonging to the triazole family, which has garnered attention for its diverse biological activities. This article provides an overview of its biological effects, mechanisms of action, and relevant case studies.
Overview of this compound
This compound is a heterocyclic compound with the molecular formula . It is known for its stability and ability to interact with various biological macromolecules, making it a subject of interest in medicinal chemistry and pharmacology.
The biological activity of this compound can be attributed to several mechanisms:
- Enzyme Inhibition : Triazoles can inhibit enzymes such as acetylcholinesterase (AChE) and butyrylcholinesterase (BuChE), which are important for neurotransmitter regulation.
- Anticancer Effects : The compound has been shown to inhibit various enzymes involved in cancer progression, including carbonic anhydrases and vascular endothelial growth factor receptors.
- Antimicrobial Activity : Research indicates that this compound exhibits significant antimicrobial properties against both Gram-positive and Gram-negative bacteria .
Antimicrobial Properties
This compound has demonstrated notable antibacterial activity. Various studies have reported its effectiveness against multiple bacterial strains:
Bacterial Strain | Activity (MIC) | Reference |
---|---|---|
Staphylococcus aureus | 10 µg/mL | |
Escherichia coli | 15 µg/mL | |
Pseudomonas aeruginosa | 20 µg/mL |
Anticancer Activity
Research has shown that this compound can inhibit the growth of cancer cells. In vitro studies indicate its potential to induce apoptosis in cancer cell lines through the modulation of cytokine release:
- Cytokine Modulation : The compound influences the release of pro-inflammatory cytokines such as TNF-α and IL-6, which are critical in tumor progression .
Antioxidant Properties
The antioxidant activity of this compound has also been documented. It scavenges free radicals and reduces oxidative stress in cellular models.
Study on Antimicrobial Efficacy
A recent study evaluated the antimicrobial effects of various triazole derivatives including this compound. The results indicated that it exhibited a broader spectrum of activity compared to standard antibiotics against resistant strains like MRSA .
Toxicity Assessment
In toxicity studies involving Wistar rats, doses up to 2000 mg/kg showed no severe adverse effects; however, some behavioral changes were noted at higher concentrations. The NOAEL (No Observed Adverse Effect Level) was established at <100 mg/kg based on observed effects on body weight and placental development .
Q & A
Basic Research Questions
Q. What are the standard synthetic routes for 3-ethyl-1H-1,2,4-triazole, and how can reaction conditions be optimized for yield?
The synthesis of this compound typically involves cyclocondensation reactions. A reported method (56% yield) uses formic hydrazide and thiopropionamide heated at 150°C under nitrogen, followed by purification via column chromatography . Key factors for optimization include temperature control, inert atmosphere, and solvent selection (e.g., ethyl acetate/CH₂Cl₂ mixtures). Monitoring reaction progress with NMR and mass spectrometry ensures intermediate validation .
Q. How is the molecular structure of this compound derivatives confirmed experimentally?
Single-crystal X-ray diffraction (SC-XRD) is the gold standard for structural elucidation. For example, the crystal structure of this compound-5(4H)-thione revealed a planar molecule with N–H···N/S hydrogen bonding and π-π stacking (3.376 Å interplanar distance) . Refinement using SHELX software (e.g., SHELXL97) ensures accuracy, with R-factors <0.05 indicating high reliability . Spectroscopic techniques (IR, ¹H/¹³C NMR) and elemental analysis complement structural validation .
Q. What pharmacological targets are commonly associated with 1,2,4-triazole derivatives?
Triazole derivatives exhibit antibacterial, antifungal, and antioxidant activities. For instance, molecular docking studies on this compound analogs against 14α-demethylase lanosterol (PDB: 3LD6) suggest antifungal potential by inhibiting ergosterol biosynthesis . In vitro assays using microbial strains (e.g., Candida albicans) and cytotoxicity screening (e.g., MTT assays) are standard for bioactivity evaluation .
Advanced Research Questions
Q. How can researchers resolve contradictions in crystallographic data for triazole derivatives?
Discrepancies in bond lengths or hydrogen bonding patterns may arise from polymorphism or refinement errors. Cross-validation with spectroscopic data (e.g., IR frequencies for N–H stretches) and computational geometry optimization (DFT calculations) can clarify ambiguities . For example, the sulfur vs. oxygen occupancy in thione/keto tautomers requires careful analysis of electron density maps and Hirshfeld surface analysis .
Q. What strategies improve synthetic yields of this compound in large-scale reactions?
Scalability challenges include side reactions (e.g., dimerization) and purification inefficiencies. Flow chemistry systems enhance heat/mass transfer, while microwave-assisted synthesis reduces reaction time . Post-synthetic modifications (e.g., Schiff base formation with aldehydes) can stabilize intermediates, as seen in 4-amino-3,5-bis(2,4-dichlorophenoxy)-1,2,4-triazole synthesis .
Q. How do computational methods enhance the prediction of triazole derivative bioactivity?
Molecular docking (AutoDock Vina, Glide) and MD simulations predict binding affinities and stability. For example, docking scores for this compound analogs correlated with experimental IC₅₀ values against fungal targets . QSAR models using descriptors like LogP and TPSA (41.57 Ų for this compound) optimize pharmacokinetic properties .
Q. What experimental designs address stability issues in triazole-based energetic materials?
For compounds like fluorodinitromethyl-triazoles, stability is assessed via thermal analysis (DSC/TGA) and impact sensitivity testing. Crystal engineering (e.g., π-π interactions in this compound-5(4H)-thione) enhances stability, while Kamlet-Jacobs equations predict detonation velocity (e.g., ~8,500 m/s for FDNMT) .
Q. Methodological Notes
- Synthesis : Prioritize inert conditions and real-time monitoring (e.g., in situ FTIR) to avoid byproducts .
- Structural Analysis : Combine SC-XRD with Hirshfeld surfaces to quantify intermolecular interactions .
- Bioactivity Screening : Use high-throughput assays (e.g., microdilution for MIC determination) and validate with in vivo models .
Properties
IUPAC Name |
5-ethyl-1H-1,2,4-triazole | |
---|---|---|
Source | PubChem | |
URL | https://pubchem.ncbi.nlm.nih.gov | |
Description | Data deposited in or computed by PubChem | |
InChI |
InChI=1S/C4H7N3/c1-2-4-5-3-6-7-4/h3H,2H2,1H3,(H,5,6,7) | |
Source | PubChem | |
URL | https://pubchem.ncbi.nlm.nih.gov | |
Description | Data deposited in or computed by PubChem | |
InChI Key |
JDIPHBYZUMQFQV-UHFFFAOYSA-N | |
Source | PubChem | |
URL | https://pubchem.ncbi.nlm.nih.gov | |
Description | Data deposited in or computed by PubChem | |
Canonical SMILES |
CCC1=NC=NN1 | |
Source | PubChem | |
URL | https://pubchem.ncbi.nlm.nih.gov | |
Description | Data deposited in or computed by PubChem | |
Molecular Formula |
C4H7N3 | |
Source | PubChem | |
URL | https://pubchem.ncbi.nlm.nih.gov | |
Description | Data deposited in or computed by PubChem | |
DSSTOX Substance ID |
DTXSID40225058 | |
Record name | 3-Ethyl-1,2,4(1H)-triazole | |
Source | EPA DSSTox | |
URL | https://comptox.epa.gov/dashboard/DTXSID40225058 | |
Description | DSSTox provides a high quality public chemistry resource for supporting improved predictive toxicology. | |
Molecular Weight |
97.12 g/mol | |
Source | PubChem | |
URL | https://pubchem.ncbi.nlm.nih.gov | |
Description | Data deposited in or computed by PubChem | |
CAS No. |
7411-16-7 | |
Record name | 3-Ethyl-1,2,4(1H)-triazole | |
Source | ChemIDplus | |
URL | https://pubchem.ncbi.nlm.nih.gov/substance/?source=chemidplus&sourceid=0007411167 | |
Description | ChemIDplus is a free, web search system that provides access to the structure and nomenclature authority files used for the identification of chemical substances cited in National Library of Medicine (NLM) databases, including the TOXNET system. | |
Record name | 3-Ethyl-1,2,4(1H)-triazole | |
Source | EPA DSSTox | |
URL | https://comptox.epa.gov/dashboard/DTXSID40225058 | |
Description | DSSTox provides a high quality public chemistry resource for supporting improved predictive toxicology. | |
Retrosynthesis Analysis
AI-Powered Synthesis Planning: Our tool employs the Template_relevance Pistachio, Template_relevance Bkms_metabolic, Template_relevance Pistachio_ringbreaker, Template_relevance Reaxys, Template_relevance Reaxys_biocatalysis model, leveraging a vast database of chemical reactions to predict feasible synthetic routes.
One-Step Synthesis Focus: Specifically designed for one-step synthesis, it provides concise and direct routes for your target compounds, streamlining the synthesis process.
Accurate Predictions: Utilizing the extensive PISTACHIO, BKMS_METABOLIC, PISTACHIO_RINGBREAKER, REAXYS, REAXYS_BIOCATALYSIS database, our tool offers high-accuracy predictions, reflecting the latest in chemical research and data.
Strategy Settings
Precursor scoring | Relevance Heuristic |
---|---|
Min. plausibility | 0.01 |
Model | Template_relevance |
Template Set | Pistachio/Bkms_metabolic/Pistachio_ringbreaker/Reaxys/Reaxys_biocatalysis |
Top-N result to add to graph | 6 |
Feasible Synthetic Routes
Disclaimer and Information on In-Vitro Research Products
Please be aware that all articles and product information presented on BenchChem are intended solely for informational purposes. The products available for purchase on BenchChem are specifically designed for in-vitro studies, which are conducted outside of living organisms. In-vitro studies, derived from the Latin term "in glass," involve experiments performed in controlled laboratory settings using cells or tissues. It is important to note that these products are not categorized as medicines or drugs, and they have not received approval from the FDA for the prevention, treatment, or cure of any medical condition, ailment, or disease. We must emphasize that any form of bodily introduction of these products into humans or animals is strictly prohibited by law. It is essential to adhere to these guidelines to ensure compliance with legal and ethical standards in research and experimentation.