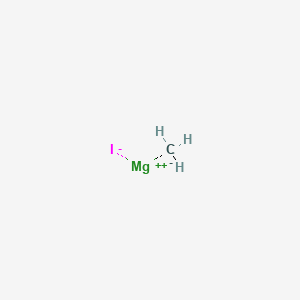
Methylmagnesium Iodide
Overview
Description
Methylmagnesium iodide is an organomagnesium compound and a type of Grignard reagent. It is represented by the chemical formula CH₃MgI. This compound is widely used in organic synthesis due to its ability to form carbon-carbon bonds, making it a valuable tool in the creation of complex molecules.
Mechanism of Action
Target of Action
Methylmagnesium iodide, with the chemical formula CH3MgI, is an organometallic compound . It is a Grignard reagent, named after its discoverer, Francois Auguste Victor Grignard, who received the 1912 Nobel Prize in Chemistry . The primary targets of this compound are organic compounds, particularly those containing carbonyl groups . It is known to target the central nervous system .
Mode of Action
This compound is a strong nucleophile and base . The carbon–magnesium bond in this compound is polarized, making the carbon atom both nucleophilic and basic . This allows it to participate in various reactions, including Grignard reactions, where it can add to carbonyl groups to form alcohols .
Biochemical Pathways
This compound is primarily used in laboratory settings for synthetic reactions . It is involved in the preparation of a variety of organic compounds, including cis-1,2-dialkylcyclopropanols, 2,4-disubstituted selenochromenes, and 7β-methyl-substituted 5-androstene derivatives . These reactions often involve the formation of new carbon-carbon bonds, enabling the synthesis of complex organic molecules .
Result of Action
The result of this compound’s action is the formation of new organic compounds. For example, it can react with carbonyl compounds to form alcohols . It can also participate in cross-coupling reactions, such as the Kumada cross-coupling, to form new carbon-carbon bonds .
Action Environment
This compound is sensitive to moisture and must be protected from atmospheric moisture to prevent it from being protonated and destroyed in acid–base reactions . It is typically used in anhydrous conditions and often in solvents like diethyl ether or tetrahydrofuran . It is also sensitive to heat, and thermal decomposition can lead to the release of irritating gases and vapors . Therefore, the environment significantly influences the action, efficacy, and stability of this compound.
Biochemical Analysis
Biochemical Properties
Methylmagnesium Iodide is a strong nucleophile and base . It is known to react with carbonyl compounds, forming new carbon-carbon bonds . This property makes it a valuable tool in the synthesis of complex organic molecules .
Cellular Effects
While this compound itself does not occur in living organisms, it serves as a useful model for other, more complex carbon-based nucleophiles that are important in biological chemistry
Molecular Mechanism
The molecular mechanism of this compound involves the addition of a carbanion nucleophile to a carbonyl compound . This reaction results in the formation of a new carbon-carbon bond . The carbon-magnesium bond in this compound is polarized, making the carbon atom both nucleophilic and basic .
Temporal Effects in Laboratory Settings
This compound is highly reactive and sensitive to moisture . It must be handled under inert gas and protected from moisture to prevent degradation
Dosage Effects in Animal Models
Iodine-containing products, which include compounds like this compound, should not be administered to pregnant, hyperthyroid, or milk-producing animals .
Metabolic Pathways
This compound does not naturally occur in biological systems and is not involved in any known metabolic pathways. It serves as a model for other carbon-based nucleophiles that play important roles in various metabolic pathways .
Subcellular Localization
The subcellular localization of this compound is not well-studied. Given its reactivity, it is unlikely to be stable within cellular compartments. The localization of similar carbon-based nucleophiles could provide insights into potential subcellular targets of this compound .
Preparation Methods
Synthetic Routes and Reaction Conditions: Methylmagnesium iodide is typically prepared by reacting methyl iodide with magnesium metal in an anhydrous ether solvent. The reaction is carried out under an inert atmosphere to prevent the Grignard reagent from reacting with moisture or oxygen. The general reaction is as follows:
CH3I+Mg→CH3MgI
Industrial Production Methods: In industrial settings, the preparation of this compound follows similar principles but on a larger scale. The reaction is conducted in large reactors with precise control over temperature and pressure to ensure high yields and purity of the product.
Chemical Reactions Analysis
Types of Reactions: Methylmagnesium iodide undergoes several types of reactions, including:
Addition Reactions: It adds to carbonyl compounds (aldehydes, ketones, esters) to form alcohols.
Substitution Reactions: It can replace halogens in organic halides.
Reduction Reactions: It can reduce certain compounds by donating electrons.
Common Reagents and Conditions:
Carbonyl Compounds: Reacts with aldehydes and ketones to form secondary and tertiary alcohols, respectively.
Esters and Acyl Halides: Reacts to form tertiary alcohols.
Epoxides: Opens the epoxide ring to form alcohols.
Major Products Formed:
Primary Alcohols: From reaction with formaldehyde.
Secondary Alcohols: From reaction with other aldehydes.
Tertiary Alcohols: From reaction with ketones, esters, and acyl halides.
Scientific Research Applications
Methylmagnesium iodide is extensively used in scientific research for:
Organic Synthesis: It is a key reagent in the formation of carbon-carbon bonds, essential for building complex organic molecules.
Pharmaceuticals: Used in the synthesis of various pharmaceutical intermediates.
Material Science: Utilized in the preparation of advanced materials and polymers.
Biological Studies: Employed in the synthesis of biologically active compounds for research purposes.
Comparison with Similar Compounds
- Methylmagnesium Bromide (CH₃MgBr)
- Methylmagnesium Chloride (CH₃MgCl)
- Methyllithium (CH₃Li)
Comparison:
- Reactivity: Methylmagnesium iodide is generally more reactive than its bromide and chloride counterparts due to the weaker carbon-iodine bond.
- Stability: Methyllithium is more reactive than this compound but less stable and more difficult to handle.
- Applications: While all these compounds are used in similar types of reactions, the choice of reagent often depends on the specific reaction conditions and desired outcomes.
This compound stands out due to its balance of reactivity and stability, making it a versatile reagent in organic synthesis.
Properties
IUPAC Name |
magnesium;carbanide;iodide | |
---|---|---|
Source | PubChem | |
URL | https://pubchem.ncbi.nlm.nih.gov | |
Description | Data deposited in or computed by PubChem | |
InChI |
InChI=1S/CH3.HI.Mg/h1H3;1H;/q-1;;+2/p-1 | |
Source | PubChem | |
URL | https://pubchem.ncbi.nlm.nih.gov | |
Description | Data deposited in or computed by PubChem | |
InChI Key |
VXWPONVCMVLXBW-UHFFFAOYSA-M | |
Source | PubChem | |
URL | https://pubchem.ncbi.nlm.nih.gov | |
Description | Data deposited in or computed by PubChem | |
Canonical SMILES |
[CH3-].[Mg+2].[I-] | |
Source | PubChem | |
URL | https://pubchem.ncbi.nlm.nih.gov | |
Description | Data deposited in or computed by PubChem | |
Molecular Formula |
CH3IMg | |
Source | PubChem | |
URL | https://pubchem.ncbi.nlm.nih.gov | |
Description | Data deposited in or computed by PubChem | |
DSSTOX Substance ID |
DTXSID301015526 | |
Record name | Iodomethylmagnesium | |
Source | EPA DSSTox | |
URL | https://comptox.epa.gov/dashboard/DTXSID301015526 | |
Description | DSSTox provides a high quality public chemistry resource for supporting improved predictive toxicology. | |
Molecular Weight |
166.24 g/mol | |
Source | PubChem | |
URL | https://pubchem.ncbi.nlm.nih.gov | |
Description | Data deposited in or computed by PubChem | |
CAS No. |
917-64-6 | |
Record name | Magnesium, iodomethyl- | |
Source | ChemIDplus | |
URL | https://pubchem.ncbi.nlm.nih.gov/substance/?source=chemidplus&sourceid=0000917646 | |
Description | ChemIDplus is a free, web search system that provides access to the structure and nomenclature authority files used for the identification of chemical substances cited in National Library of Medicine (NLM) databases, including the TOXNET system. | |
Record name | Magnesium, iodomethyl- | |
Source | EPA Chemicals under the TSCA | |
URL | https://www.epa.gov/chemicals-under-tsca | |
Description | EPA Chemicals under the Toxic Substances Control Act (TSCA) collection contains information on chemicals and their regulations under TSCA, including non-confidential content from the TSCA Chemical Substance Inventory and Chemical Data Reporting. | |
Record name | Iodomethylmagnesium | |
Source | EPA DSSTox | |
URL | https://comptox.epa.gov/dashboard/DTXSID301015526 | |
Description | DSSTox provides a high quality public chemistry resource for supporting improved predictive toxicology. | |
Record name | Iodomethylmagnesium | |
Source | European Chemicals Agency (ECHA) | |
URL | https://echa.europa.eu/substance-information/-/substanceinfo/100.011.847 | |
Description | The European Chemicals Agency (ECHA) is an agency of the European Union which is the driving force among regulatory authorities in implementing the EU's groundbreaking chemicals legislation for the benefit of human health and the environment as well as for innovation and competitiveness. | |
Explanation | Use of the information, documents and data from the ECHA website is subject to the terms and conditions of this Legal Notice, and subject to other binding limitations provided for under applicable law, the information, documents and data made available on the ECHA website may be reproduced, distributed and/or used, totally or in part, for non-commercial purposes provided that ECHA is acknowledged as the source: "Source: European Chemicals Agency, http://echa.europa.eu/". Such acknowledgement must be included in each copy of the material. ECHA permits and encourages organisations and individuals to create links to the ECHA website under the following cumulative conditions: Links can only be made to webpages that provide a link to the Legal Notice page. | |
Q1: How does methylmagnesium iodide react with ketones?
A1: this compound adds to the carbonyl group of ketones, forming a new carbon-carbon bond and generating a tertiary alcohol upon hydrolysis. [, , , ]
Q2: Can this compound react with other functional groups besides ketones?
A2: Yes, this compound can react with a variety of functional groups including aldehydes, esters, nitriles, epoxides, and even some activated halides. [, , , , , , ]
Q3: Are there examples of unexpected reactions with this compound?
A3: Yes, some reactions deviate from typical Grignard additions. For example, this compound can induce ring-opening reactions with certain cyclic diones [] or promote unusual methyl insertion reactions with specific spiro[cyclohexan-1,3'-3'H-indole] derivatives. []
Q4: What is the molecular formula and weight of this compound?
A4: The molecular formula is CH3MgI, and the molecular weight is 142.32 g/mol.
Q5: Is there spectroscopic data available for this compound?
A5: While the provided articles don't present specific spectroscopic data for CH3MgI itself, they often characterize the products formed in reactions with this compound using techniques like infrared (IR) spectroscopy, nuclear magnetic resonance (NMR) spectroscopy, and mass spectrometry (MS). [, , , , ]
Q6: In what solvents is this compound typically prepared and used?
A6: this compound is typically prepared and used in ethereal solvents like diethyl ether, tetrahydrofuran (THF), or dimethoxyethane (DME). [, , , , ]
Q7: What are the stability considerations for this compound?
A7: this compound is highly sensitive to moisture and air, reacting vigorously with water and oxygen. It should be handled under an inert atmosphere, such as argon or nitrogen, and used immediately after preparation. [, ]
Q8: Is this compound used as a catalyst in any of the mentioned research?
A8: The provided research primarily employs this compound as a stoichiometric reagent rather than a catalyst.
Q9: Can you elaborate on the role of this compound in the synthesis of (±)-cannabidiol?
A9: In the synthesis of (±)-cannabidiol, this compound is crucial for the demethylation of (±)-cannabidiol dimethyl ether, achieved by heating the ether with an excess of dry this compound at elevated temperatures. This demethylation method proved vital in overcoming the limitations of traditional acidic or basic reagents. []
Q10: Is computational chemistry used to study this compound or its reactions?
A10: The provided articles primarily focus on experimental aspects. While they don't delve into computational studies on this compound itself, they occasionally utilize X-ray diffraction analysis to determine the structures of complex reaction products. [, , ]
Q11: How does modifying the Grignard reagent, by using a different alkyl or aryl group instead of methyl, influence the reaction outcome?
A11: Changing the Grignard reagent can significantly impact the reaction. For instance, using phenylmagnesium bromide instead of this compound with certain halotropolone methyl ethers leads to the formation of different products, including substituted tropones and benzophenones. []
Q12: Are there specific formulation strategies mentioned for this compound?
A12: The articles primarily focus on synthetic applications and don't delve into specific formulation strategies for this compound.
Q13: Are there specific recommendations for handling waste containing this compound?
A13: this compound is highly reactive and should be quenched carefully following established safety protocols. Waste containing Grignard reagents should be handled and disposed of according to local regulations and guidelines for hazardous waste.
Q14: What is the historical significance of Grignard reagents like this compound in organic chemistry?
A15: The discovery of Grignard reagents in the early 20th century revolutionized organic synthesis. Their ability to form new carbon-carbon bonds under relatively mild conditions opened up vast possibilities for constructing complex molecules. [, ]
Q15: Are there cross-disciplinary applications of this compound or its reaction products?
A16: While the provided articles focus on synthetic chemistry, the resulting molecules from reactions with this compound can have applications in various fields. For example, the synthesis of (±)-cannabidiol, a significant component of cannabis with potential therapeutic properties, highlights the broader impact of Grignard chemistry. []
Disclaimer and Information on In-Vitro Research Products
Please be aware that all articles and product information presented on BenchChem are intended solely for informational purposes. The products available for purchase on BenchChem are specifically designed for in-vitro studies, which are conducted outside of living organisms. In-vitro studies, derived from the Latin term "in glass," involve experiments performed in controlled laboratory settings using cells or tissues. It is important to note that these products are not categorized as medicines or drugs, and they have not received approval from the FDA for the prevention, treatment, or cure of any medical condition, ailment, or disease. We must emphasize that any form of bodily introduction of these products into humans or animals is strictly prohibited by law. It is essential to adhere to these guidelines to ensure compliance with legal and ethical standards in research and experimentation.