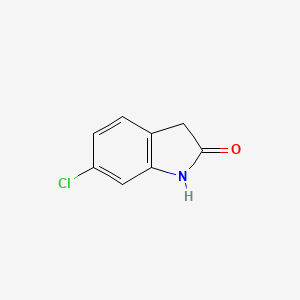
6-Chlorooxindole
Overview
Description
6-Chlorooxindole, also known as 6-chloro-1,3-dihydroindol-2-one, is a chemical compound with the molecular formula C8H6ClNO and a molecular weight of 167.59 g/mol . It is a derivative of oxindole, characterized by the presence of a chlorine atom at the sixth position of the indole ring. This compound is known for its applications in various fields, including medicinal chemistry and organic synthesis.
Preparation Methods
Synthetic Routes and Reaction Conditions: The synthesis of 6-Chlorooxindole typically involves the following steps:
Starting Material: The process begins with 4-chloro-2-nitrotoluene.
Formation of 3-(2-nitro-4-chlorophenyl)pyruvic acid: This is achieved by reacting 4-chloro-2-nitrotoluene with sodium ethoxide and diethyl oxalate in ethanol, followed by refluxing with hydrogen peroxide and acidification.
Formation of 4-chloro-2-nitrophenylacetic acid: The intermediate is treated with sodium hydroxide and hydrogen peroxide, followed by acidification to yield 4-chloro-2-nitrophenylacetic acid.
Reductive Cyclization: The final step involves reductive cyclization of 4-chloro-2-nitrophenylacetic acid to produce this compound.
Industrial Production Methods: Industrial production methods for this compound are similar to laboratory synthesis but are scaled up to accommodate larger quantities. The process involves the same starting materials and reaction conditions, with optimizations for yield and purity.
Chemical Reactions Analysis
Types of Reactions: 6-Chlorooxindole undergoes various chemical reactions, including:
Oxidation: It can be oxidized to form corresponding oxindole derivatives.
Reduction: Reduction reactions can convert it to different reduced forms of oxindole.
Substitution: The chlorine atom can be substituted with other functional groups under appropriate conditions.
Common Reagents and Conditions:
Oxidation: Common oxidizing agents include hydrogen peroxide and other peroxides.
Reduction: Reducing agents such as sodium borohydride or lithium aluminum hydride are used.
Substitution: Reagents like sodium ethoxide and diethyl oxalate are employed for substitution reactions.
Major Products Formed: The major products formed from these reactions include various substituted oxindole derivatives, which have significant applications in medicinal chemistry .
Scientific Research Applications
6-Chlorooxindole has a wide range of applications in scientific research:
Mechanism of Action
The mechanism of action of 6-Chlorooxindole involves its interaction with specific molecular targets and pathways:
Comparison with Similar Compounds
5-Chloro-2-oxindole: Another chlorinated oxindole with similar properties but different biological activities.
2-Oxindole: The parent compound without the chlorine substitution, used in various synthetic applications.
1-Methyl-2-oxindole: A methylated derivative with distinct chemical and biological properties.
Uniqueness of 6-Chlorooxindole: this compound is unique due to its specific substitution pattern, which imparts distinct chemical reactivity and biological activity. Its chlorine atom at the sixth position makes it a valuable intermediate in the synthesis of various pharmaceuticals and industrial chemicals .
Properties
IUPAC Name |
6-chloro-1,3-dihydroindol-2-one | |
---|---|---|
Source | PubChem | |
URL | https://pubchem.ncbi.nlm.nih.gov | |
Description | Data deposited in or computed by PubChem | |
InChI |
InChI=1S/C8H6ClNO/c9-6-2-1-5-3-8(11)10-7(5)4-6/h1-2,4H,3H2,(H,10,11) | |
Source | PubChem | |
URL | https://pubchem.ncbi.nlm.nih.gov | |
Description | Data deposited in or computed by PubChem | |
InChI Key |
CENVPIZOTHULGJ-UHFFFAOYSA-N | |
Source | PubChem | |
URL | https://pubchem.ncbi.nlm.nih.gov | |
Description | Data deposited in or computed by PubChem | |
Canonical SMILES |
C1C2=C(C=C(C=C2)Cl)NC1=O | |
Source | PubChem | |
URL | https://pubchem.ncbi.nlm.nih.gov | |
Description | Data deposited in or computed by PubChem | |
Molecular Formula |
C8H6ClNO | |
Source | PubChem | |
URL | https://pubchem.ncbi.nlm.nih.gov | |
Description | Data deposited in or computed by PubChem | |
DSSTOX Substance ID |
DTXSID00353003 | |
Record name | 6-Chlorooxindole | |
Source | EPA DSSTox | |
URL | https://comptox.epa.gov/dashboard/DTXSID00353003 | |
Description | DSSTox provides a high quality public chemistry resource for supporting improved predictive toxicology. | |
Molecular Weight |
167.59 g/mol | |
Source | PubChem | |
URL | https://pubchem.ncbi.nlm.nih.gov | |
Description | Data deposited in or computed by PubChem | |
CAS No. |
56341-37-8 | |
Record name | 6-Chlorooxindole | |
Source | CAS Common Chemistry | |
URL | https://commonchemistry.cas.org/detail?cas_rn=56341-37-8 | |
Description | CAS Common Chemistry is an open community resource for accessing chemical information. Nearly 500,000 chemical substances from CAS REGISTRY cover areas of community interest, including common and frequently regulated chemicals, and those relevant to high school and undergraduate chemistry classes. This chemical information, curated by our expert scientists, is provided in alignment with our mission as a division of the American Chemical Society. | |
Explanation | The data from CAS Common Chemistry is provided under a CC-BY-NC 4.0 license, unless otherwise stated. | |
Record name | 6-Chlorooxindole | |
Source | EPA DSSTox | |
URL | https://comptox.epa.gov/dashboard/DTXSID00353003 | |
Description | DSSTox provides a high quality public chemistry resource for supporting improved predictive toxicology. | |
Record name | 6-chloro-1,3-dihydroindol-2-one | |
Source | European Chemicals Agency (ECHA) | |
URL | https://echa.europa.eu/substance-information/-/substanceinfo/100.101.992 | |
Description | The European Chemicals Agency (ECHA) is an agency of the European Union which is the driving force among regulatory authorities in implementing the EU's groundbreaking chemicals legislation for the benefit of human health and the environment as well as for innovation and competitiveness. | |
Explanation | Use of the information, documents and data from the ECHA website is subject to the terms and conditions of this Legal Notice, and subject to other binding limitations provided for under applicable law, the information, documents and data made available on the ECHA website may be reproduced, distributed and/or used, totally or in part, for non-commercial purposes provided that ECHA is acknowledged as the source: "Source: European Chemicals Agency, http://echa.europa.eu/". Such acknowledgement must be included in each copy of the material. ECHA permits and encourages organisations and individuals to create links to the ECHA website under the following cumulative conditions: Links can only be made to webpages that provide a link to the Legal Notice page. | |
Record name | 2H-Indol-2-one, 6-chloro-1,3-dihydro | |
Source | European Chemicals Agency (ECHA) | |
URL | https://echa.europa.eu/substance-information/-/substanceinfo/100.107.776 | |
Description | The European Chemicals Agency (ECHA) is an agency of the European Union which is the driving force among regulatory authorities in implementing the EU's groundbreaking chemicals legislation for the benefit of human health and the environment as well as for innovation and competitiveness. | |
Explanation | Use of the information, documents and data from the ECHA website is subject to the terms and conditions of this Legal Notice, and subject to other binding limitations provided for under applicable law, the information, documents and data made available on the ECHA website may be reproduced, distributed and/or used, totally or in part, for non-commercial purposes provided that ECHA is acknowledged as the source: "Source: European Chemicals Agency, http://echa.europa.eu/". Such acknowledgement must be included in each copy of the material. ECHA permits and encourages organisations and individuals to create links to the ECHA website under the following cumulative conditions: Links can only be made to webpages that provide a link to the Legal Notice page. | |
Synthesis routes and methods I
Procedure details
Synthesis routes and methods II
Procedure details
Synthesis routes and methods III
Procedure details
Retrosynthesis Analysis
AI-Powered Synthesis Planning: Our tool employs the Template_relevance Pistachio, Template_relevance Bkms_metabolic, Template_relevance Pistachio_ringbreaker, Template_relevance Reaxys, Template_relevance Reaxys_biocatalysis model, leveraging a vast database of chemical reactions to predict feasible synthetic routes.
One-Step Synthesis Focus: Specifically designed for one-step synthesis, it provides concise and direct routes for your target compounds, streamlining the synthesis process.
Accurate Predictions: Utilizing the extensive PISTACHIO, BKMS_METABOLIC, PISTACHIO_RINGBREAKER, REAXYS, REAXYS_BIOCATALYSIS database, our tool offers high-accuracy predictions, reflecting the latest in chemical research and data.
Strategy Settings
Precursor scoring | Relevance Heuristic |
---|---|
Min. plausibility | 0.01 |
Model | Template_relevance |
Template Set | Pistachio/Bkms_metabolic/Pistachio_ringbreaker/Reaxys/Reaxys_biocatalysis |
Top-N result to add to graph | 6 |
Feasible Synthetic Routes
Disclaimer and Information on In-Vitro Research Products
Please be aware that all articles and product information presented on BenchChem are intended solely for informational purposes. The products available for purchase on BenchChem are specifically designed for in-vitro studies, which are conducted outside of living organisms. In-vitro studies, derived from the Latin term "in glass," involve experiments performed in controlled laboratory settings using cells or tissues. It is important to note that these products are not categorized as medicines or drugs, and they have not received approval from the FDA for the prevention, treatment, or cure of any medical condition, ailment, or disease. We must emphasize that any form of bodily introduction of these products into humans or animals is strictly prohibited by law. It is essential to adhere to these guidelines to ensure compliance with legal and ethical standards in research and experimentation.