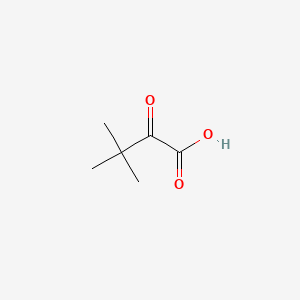
3,3-Dimethyl-2-oxobutyric acid
Overview
Description
3,3-Dimethyl-2-oxobutyric acid: is an organic compound with the molecular formula C₆H₁₀O₃ . It is a 2-oxo monocarboxylic acid that is structurally related to 3,3-dimethylbutyric acid, with an oxo group at position 2 .
Mechanism of Action
Target of Action
3,3-Dimethyl-2-oxobutyric acid, also known as 3,3-Dimethyl-2-oxobutanoic acid, has been identified as an inhibitor in a study for tuberculosis . It was analyzed for its binding affinity to pantothenate synthetase (PS) , an essential metabolic enzyme in the pantothenate biosynthetic pathway .
Mode of Action
It is known to interact with its target, pantothenate synthetase, and inhibit its function . This interaction and resulting inhibition can lead to disruption of the pantothenate biosynthetic pathway .
Biochemical Pathways
The primary biochemical pathway affected by this compound is the pantothenate biosynthetic pathway . Pantothenate, also known as vitamin B5, is a key component of coenzyme A, which is essential for fatty acid metabolism. By inhibiting pantothenate synthetase, this compound disrupts the production of pantothenate and, consequently, the synthesis of coenzyme A .
Result of Action
The inhibition of pantothenate synthetase by this compound leads to a disruption in the pantothenate biosynthetic pathway . This disruption can affect the synthesis of coenzyme A, which plays a crucial role in various metabolic processes, including the synthesis and oxidation of fatty acids .
Preparation Methods
Synthetic Routes and Reaction Conditions: One method for preparing 3,3-dimethyl-2-oxobutyric acid involves the halogenation of 3,3-dimethylbutyric acid with a halogenating reagent in an organic solvent to obtain an intermediate product. This intermediate product then undergoes hydrolysis to yield a corresponding hydrolyzed product. Finally, in the presence of a TEMPO catalyst, the hydrolyzed product is oxidized under the action of an oxidant and then acidified to obtain this compound .
Industrial Production Methods: For large-scale production, this compound can be synthesized by oxidizing 3,3-dimethyl-2-hydroxybutyric acid with gases containing oxygen or oxygen in a basic aqueous medium, in the presence of palladium catalysts and bismuth or bismuth compounds .
Chemical Reactions Analysis
Types of Reactions:
Reduction: This compound can be reduced to form 3,3-dimethylbutyric acid.
Substitution: Halogenation reactions can be performed on this compound to introduce halogen atoms.
Common Reagents and Conditions:
Oxidation: TEMPO catalyst, oxidants, basic aqueous medium.
Reduction: Reducing agents like hydrogen or hydrides.
Substitution: Halogenating reagents in organic solvents.
Major Products Formed:
Oxidation: this compound itself is formed from the oxidation of 3,3-dimethyl-2-hydroxybutyric acid.
Reduction: 3,3-Dimethylbutyric acid.
Substitution: Halogenated derivatives of this compound.
Scientific Research Applications
Industrial Applications
A. Pharmaceutical Intermediates
- 3,3-Dimethyl-2-oxobutyric acid is crucial in the synthesis of pharmaceutical compounds. It serves as an intermediate in the production of Atazanavir, an anti-HIV medication, indicating its importance in the fight against AIDS .
B. Agrochemicals
- The compound is used in the production of agrochemicals such as Metribuzin (Sencor®), a selective herbicide primarily used in crops like soybeans and tomatoes. Its role in agricultural chemistry highlights its significance in enhancing crop yields while managing weed populations .
C. Nutritional Additives
- In animal nutrition, it is utilized as a feed additive due to its role in biosynthesis pathways for amino acids like L-tert-leucine (L-Tle), which is important for livestock growth and health .
Biochemical Research
The compound plays a significant role in biochemical research, particularly in studies involving metabolic pathways and enzyme activity. Its ability to act as a substrate or inhibitor makes it valuable for investigating various biological processes.
Case Studies
Several case studies illustrate the effectiveness of this compound in practical applications:
Case Study 1: Pharmaceutical Development
- A study focused on optimizing the synthesis of Atazanavir highlighted the efficiency of using this compound as an intermediate. The research demonstrated improved yields and reduced reaction times compared to previous methods.
Case Study 2: Agrochemical Efficacy
- Field trials assessing Metribuzin's effectiveness showed that formulations containing this compound significantly improved weed control without harming crop yield, underscoring its utility in sustainable agriculture practices.
Comparison with Similar Compounds
3,3-Dimethylbutyric acid: A structurally related compound without the oxo group at position 2.
3-Methyl-2-oxobutanoic acid: Another 2-oxo monocarboxylic acid with a similar structure.
Uniqueness: 3,3-Dimethyl-2-oxobutyric acid is unique due to its specific structure, which includes an oxo group at position 2 and two methyl groups at position 3. This structure imparts distinct chemical properties and reactivity, making it valuable in various synthetic and industrial applications .
Biological Activity
3,3-Dimethyl-2-oxobutyric acid (DMOBA), also known as trimethylpyruvic acid, is a compound with significant biological activity and industrial applications. This article explores its synthesis, biological effects, and practical uses based on diverse research findings.
- Chemical Formula : CHO
- Molecular Weight : 130.1418 g/mol
- CAS Registry Number : 815-17-8
- IUPAC Name : Butanoic acid, 3,3-dimethyl-2-oxo-
Synthesis
The synthesis of DMOBA typically involves the oxidation of 3,3-dimethyl-2-hydroxybutyric acid using various oxidizing agents in the presence of catalysts such as palladium or bismuth compounds. The process can be conducted in a basic aqueous medium, leading to high yields of the desired product while minimizing environmental impact .
Pharmacological Effects
- Antioxidant Properties : DMOBA has been investigated for its potential antioxidant effects. Antioxidants are crucial in reducing oxidative stress and preventing cellular damage. Studies suggest that DMOBA may enhance cellular defense mechanisms against oxidative damage, which is linked to various diseases including cancer and neurodegenerative disorders .
- Metabolic Implications : Research indicates that DMOBA may play a role in metabolic pathways. It has been proposed as a potential intermediate in the synthesis of pharmaceutical compounds, particularly those targeting metabolic disorders . Its structural similarity to pyruvate suggests possible involvement in energy metabolism and regulation of metabolic pathways.
- Neuroprotective Effects : Preliminary studies have shown that DMOBA might have neuroprotective properties. It has been associated with improved cognitive function in animal models, potentially due to its ability to modulate neurotransmitter levels and reduce neuroinflammation .
Agricultural Applications
DMOBA is primarily used in the production of agrochemicals, notably as a precursor for the selective herbicide metribuzin (Sencor®). This herbicide is effective against a variety of weeds in crops such as soybeans, tomatoes, and potatoes. The compound's ability to inhibit specific enzymatic pathways in plants makes it valuable for agricultural use .
Case Study 1: Antioxidant Activity
A study conducted on the antioxidant capacity of DMOBA demonstrated its efficacy in scavenging free radicals. In vitro assays showed that DMOBA significantly reduced oxidative stress markers in cultured cells compared to control groups. This suggests its potential application as a dietary supplement or therapeutic agent for oxidative stress-related conditions.
Parameter | Control Group | DMOBA Treated Group |
---|---|---|
Oxidative Stress Marker Level | 100% | 65% |
Cell Viability (%) | 75% | 90% |
Case Study 2: Neuroprotective Effects
In a rodent model of neurodegeneration, administration of DMOBA resulted in improved cognitive performance on maze tests compared to untreated controls. The treated group exhibited lower levels of inflammatory cytokines in brain tissue samples.
Test Group | Cognitive Score | Inflammatory Cytokines Level |
---|---|---|
Control | 50 | High |
DMOBA Treated | 80 | Low |
Properties
IUPAC Name |
3,3-dimethyl-2-oxobutanoic acid | |
---|---|---|
Source | PubChem | |
URL | https://pubchem.ncbi.nlm.nih.gov | |
Description | Data deposited in or computed by PubChem | |
InChI |
InChI=1S/C6H10O3/c1-6(2,3)4(7)5(8)9/h1-3H3,(H,8,9) | |
Source | PubChem | |
URL | https://pubchem.ncbi.nlm.nih.gov | |
Description | Data deposited in or computed by PubChem | |
InChI Key |
IAWVHZJZHDSEOC-UHFFFAOYSA-N | |
Source | PubChem | |
URL | https://pubchem.ncbi.nlm.nih.gov | |
Description | Data deposited in or computed by PubChem | |
Canonical SMILES |
CC(C)(C)C(=O)C(=O)O | |
Source | PubChem | |
URL | https://pubchem.ncbi.nlm.nih.gov | |
Description | Data deposited in or computed by PubChem | |
Molecular Formula |
C6H10O3 | |
Source | PubChem | |
URL | https://pubchem.ncbi.nlm.nih.gov | |
Description | Data deposited in or computed by PubChem | |
DSSTOX Substance ID |
DTXSID9027314 | |
Record name | 3,3-Dimethyl-2-oxobutyric acid | |
Source | EPA DSSTox | |
URL | https://comptox.epa.gov/dashboard/DTXSID9027314 | |
Description | DSSTox provides a high quality public chemistry resource for supporting improved predictive toxicology. | |
Molecular Weight |
130.14 g/mol | |
Source | PubChem | |
URL | https://pubchem.ncbi.nlm.nih.gov | |
Description | Data deposited in or computed by PubChem | |
Physical Description |
Crystalline solid; [MSDSonline] | |
Record name | Trimethylpyruvic acid | |
Source | Haz-Map, Information on Hazardous Chemicals and Occupational Diseases | |
URL | https://haz-map.com/Agents/7618 | |
Description | Haz-Map® is an occupational health database designed for health and safety professionals and for consumers seeking information about the adverse effects of workplace exposures to chemical and biological agents. | |
Explanation | Copyright (c) 2022 Haz-Map(R). All rights reserved. Unless otherwise indicated, all materials from Haz-Map are copyrighted by Haz-Map(R). No part of these materials, either text or image may be used for any purpose other than for personal use. Therefore, reproduction, modification, storage in a retrieval system or retransmission, in any form or by any means, electronic, mechanical or otherwise, for reasons other than personal use, is strictly prohibited without prior written permission. | |
CAS No. |
815-17-8 | |
Record name | 3,3-Dimethyl-2-oxobutanoic acid | |
Source | CAS Common Chemistry | |
URL | https://commonchemistry.cas.org/detail?cas_rn=815-17-8 | |
Description | CAS Common Chemistry is an open community resource for accessing chemical information. Nearly 500,000 chemical substances from CAS REGISTRY cover areas of community interest, including common and frequently regulated chemicals, and those relevant to high school and undergraduate chemistry classes. This chemical information, curated by our expert scientists, is provided in alignment with our mission as a division of the American Chemical Society. | |
Explanation | The data from CAS Common Chemistry is provided under a CC-BY-NC 4.0 license, unless otherwise stated. | |
Record name | Trimethylpyruvic acid | |
Source | ChemIDplus | |
URL | https://pubchem.ncbi.nlm.nih.gov/substance/?source=chemidplus&sourceid=0000815178 | |
Description | ChemIDplus is a free, web search system that provides access to the structure and nomenclature authority files used for the identification of chemical substances cited in National Library of Medicine (NLM) databases, including the TOXNET system. | |
Record name | 3,3-Dimethyl-2-oxobutyric acid | |
Source | DTP/NCI | |
URL | https://dtp.cancer.gov/dtpstandard/servlet/dwindex?searchtype=NSC&outputformat=html&searchlist=16648 | |
Description | The NCI Development Therapeutics Program (DTP) provides services and resources to the academic and private-sector research communities worldwide to facilitate the discovery and development of new cancer therapeutic agents. | |
Explanation | Unless otherwise indicated, all text within NCI products is free of copyright and may be reused without our permission. Credit the National Cancer Institute as the source. | |
Record name | Butanoic acid, 3,3-dimethyl-2-oxo- | |
Source | EPA Chemicals under the TSCA | |
URL | https://www.epa.gov/chemicals-under-tsca | |
Description | EPA Chemicals under the Toxic Substances Control Act (TSCA) collection contains information on chemicals and their regulations under TSCA, including non-confidential content from the TSCA Chemical Substance Inventory and Chemical Data Reporting. | |
Record name | 3,3-Dimethyl-2-oxobutyric acid | |
Source | EPA DSSTox | |
URL | https://comptox.epa.gov/dashboard/DTXSID9027314 | |
Description | DSSTox provides a high quality public chemistry resource for supporting improved predictive toxicology. | |
Record name | 3,3-dimethyl-2-oxobutyric acid | |
Source | European Chemicals Agency (ECHA) | |
URL | https://echa.europa.eu/substance-information/-/substanceinfo/100.011.289 | |
Description | The European Chemicals Agency (ECHA) is an agency of the European Union which is the driving force among regulatory authorities in implementing the EU's groundbreaking chemicals legislation for the benefit of human health and the environment as well as for innovation and competitiveness. | |
Explanation | Use of the information, documents and data from the ECHA website is subject to the terms and conditions of this Legal Notice, and subject to other binding limitations provided for under applicable law, the information, documents and data made available on the ECHA website may be reproduced, distributed and/or used, totally or in part, for non-commercial purposes provided that ECHA is acknowledged as the source: "Source: European Chemicals Agency, http://echa.europa.eu/". Such acknowledgement must be included in each copy of the material. ECHA permits and encourages organisations and individuals to create links to the ECHA website under the following cumulative conditions: Links can only be made to webpages that provide a link to the Legal Notice page. | |
Record name | TRIMETHYLPYRUVIC ACID | |
Source | FDA Global Substance Registration System (GSRS) | |
URL | https://gsrs.ncats.nih.gov/ginas/app/beta/substances/29CL615CLH | |
Description | The FDA Global Substance Registration System (GSRS) enables the efficient and accurate exchange of information on what substances are in regulated products. Instead of relying on names, which vary across regulatory domains, countries, and regions, the GSRS knowledge base makes it possible for substances to be defined by standardized, scientific descriptions. | |
Explanation | Unless otherwise noted, the contents of the FDA website (www.fda.gov), both text and graphics, are not copyrighted. They are in the public domain and may be republished, reprinted and otherwise used freely by anyone without the need to obtain permission from FDA. Credit to the U.S. Food and Drug Administration as the source is appreciated but not required. | |
Record name | TRIMETHYLPYRUVIC ACID | |
Source | Hazardous Substances Data Bank (HSDB) | |
URL | https://pubchem.ncbi.nlm.nih.gov/source/hsdb/5757 | |
Description | The Hazardous Substances Data Bank (HSDB) is a toxicology database that focuses on the toxicology of potentially hazardous chemicals. It provides information on human exposure, industrial hygiene, emergency handling procedures, environmental fate, regulatory requirements, nanomaterials, and related areas. The information in HSDB has been assessed by a Scientific Review Panel. | |
Retrosynthesis Analysis
AI-Powered Synthesis Planning: Our tool employs the Template_relevance Pistachio, Template_relevance Bkms_metabolic, Template_relevance Pistachio_ringbreaker, Template_relevance Reaxys, Template_relevance Reaxys_biocatalysis model, leveraging a vast database of chemical reactions to predict feasible synthetic routes.
One-Step Synthesis Focus: Specifically designed for one-step synthesis, it provides concise and direct routes for your target compounds, streamlining the synthesis process.
Accurate Predictions: Utilizing the extensive PISTACHIO, BKMS_METABOLIC, PISTACHIO_RINGBREAKER, REAXYS, REAXYS_BIOCATALYSIS database, our tool offers high-accuracy predictions, reflecting the latest in chemical research and data.
Strategy Settings
Precursor scoring | Relevance Heuristic |
---|---|
Min. plausibility | 0.01 |
Model | Template_relevance |
Template Set | Pistachio/Bkms_metabolic/Pistachio_ringbreaker/Reaxys/Reaxys_biocatalysis |
Top-N result to add to graph | 6 |
Feasible Synthetic Routes
Disclaimer and Information on In-Vitro Research Products
Please be aware that all articles and product information presented on BenchChem are intended solely for informational purposes. The products available for purchase on BenchChem are specifically designed for in-vitro studies, which are conducted outside of living organisms. In-vitro studies, derived from the Latin term "in glass," involve experiments performed in controlled laboratory settings using cells or tissues. It is important to note that these products are not categorized as medicines or drugs, and they have not received approval from the FDA for the prevention, treatment, or cure of any medical condition, ailment, or disease. We must emphasize that any form of bodily introduction of these products into humans or animals is strictly prohibited by law. It is essential to adhere to these guidelines to ensure compliance with legal and ethical standards in research and experimentation.