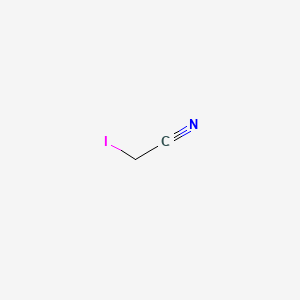
Iodoacetonitrile
Overview
Description
Iodoacetonitrile is an organic compound with the chemical formula C₂H₂IN . It is a colorless to pale yellow liquid that is used in various chemical reactions and industrial applications. The compound is known for its reactivity due to the presence of both an iodine atom and a nitrile group, making it a valuable intermediate in organic synthesis .
Preparation Methods
Synthetic Routes and Reaction Conditions: Iodoacetonitrile can be synthesized through several methods. One common method involves the reaction of acetonitrile with iodine in the presence of a base. The reaction typically proceeds as follows:
CH3CN+I2→ICH2CN+HI
Industrial Production Methods: In industrial settings, this compound is produced by the iodination of acetonitrile using iodine and a suitable catalyst. The reaction is carried out under controlled conditions to ensure high yield and purity of the product .
Chemical Reactions Analysis
Types of Reactions: Iodoacetonitrile undergoes various types of chemical reactions, including:
Substitution Reactions: It can participate in nucleophilic substitution reactions where the iodine atom is replaced by other nucleophiles.
Addition Reactions: The nitrile group can undergo addition reactions with various reagents.
Reformatsky and Wittig-type Reactions: It reacts with aldehydes to form different products
Common Reagents and Conditions:
Nucleophiles: Such as amines and thiols, are commonly used in substitution reactions.
Aldehydes: Used in Reformatsky and Wittig-type reactions.
Major Products:
Substitution Products: Depending on the nucleophile used, various substituted acetonitriles can be formed.
Addition Products: Products formed from the addition to the nitrile group
Scientific Research Applications
Iodoacetonitrile has several applications in scientific research:
Chemistry: Used as an intermediate in the synthesis of various organic compounds.
Biology: Employed in the study of enzyme mechanisms and protein modifications.
Medicine: Investigated for its potential use in drug development and as a reagent in pharmaceutical synthesis.
Industry: Utilized in the production of agrochemicals and other industrial chemicals
Mechanism of Action
The mechanism of action of iodoacetonitrile involves its reactivity with nucleophiles and electrophiles. The iodine atom in this compound is highly reactive, making it susceptible to nucleophilic attack. This reactivity is exploited in various synthetic applications to form new carbon-nitrogen and carbon-carbon bonds .
Comparison with Similar Compounds
Acetonitrile (CH₃CN): A simpler nitrile compound without the iodine atom.
Bromoacetonitrile (CH₂BrCN): Similar structure but with a bromine atom instead of iodine.
Chloroacetonitrile (CH₂ClCN): Contains a chlorine atom instead of iodine.
Uniqueness: Iodoacetonitrile is unique due to the presence of the iodine atom, which imparts distinct reactivity compared to its bromo and chloro counterparts. The iodine atom makes this compound more reactive in nucleophilic substitution reactions, allowing for a broader range of synthetic applications .
Properties
IUPAC Name |
2-iodoacetonitrile | |
---|---|---|
Source | PubChem | |
URL | https://pubchem.ncbi.nlm.nih.gov | |
Description | Data deposited in or computed by PubChem | |
InChI |
InChI=1S/C2H2IN/c3-1-2-4/h1H2 | |
Source | PubChem | |
URL | https://pubchem.ncbi.nlm.nih.gov | |
Description | Data deposited in or computed by PubChem | |
InChI Key |
VODKOOOHHCAWFR-UHFFFAOYSA-N | |
Source | PubChem | |
URL | https://pubchem.ncbi.nlm.nih.gov | |
Description | Data deposited in or computed by PubChem | |
Canonical SMILES |
C(C#N)I | |
Source | PubChem | |
URL | https://pubchem.ncbi.nlm.nih.gov | |
Description | Data deposited in or computed by PubChem | |
Molecular Formula |
C2H2IN | |
Source | PubChem | |
URL | https://pubchem.ncbi.nlm.nih.gov | |
Description | Data deposited in or computed by PubChem | |
DSSTOX Substance ID |
DTXSID8073215 | |
Record name | Acetonitrile, iodo- | |
Source | EPA DSSTox | |
URL | https://comptox.epa.gov/dashboard/DTXSID8073215 | |
Description | DSSTox provides a high quality public chemistry resource for supporting improved predictive toxicology. | |
Molecular Weight |
166.95 g/mol | |
Source | PubChem | |
URL | https://pubchem.ncbi.nlm.nih.gov | |
Description | Data deposited in or computed by PubChem | |
Physical Description |
Dark amber liquid; [MSDSonline] | |
Record name | Iodoacetonitrile | |
Source | Haz-Map, Information on Hazardous Chemicals and Occupational Diseases | |
URL | https://haz-map.com/Agents/8634 | |
Description | Haz-Map® is an occupational health database designed for health and safety professionals and for consumers seeking information about the adverse effects of workplace exposures to chemical and biological agents. | |
Explanation | Copyright (c) 2022 Haz-Map(R). All rights reserved. Unless otherwise indicated, all materials from Haz-Map are copyrighted by Haz-Map(R). No part of these materials, either text or image may be used for any purpose other than for personal use. Therefore, reproduction, modification, storage in a retrieval system or retransmission, in any form or by any means, electronic, mechanical or otherwise, for reasons other than personal use, is strictly prohibited without prior written permission. | |
CAS No. |
624-75-9 | |
Record name | Iodoacetonitrile | |
Source | CAS Common Chemistry | |
URL | https://commonchemistry.cas.org/detail?cas_rn=624-75-9 | |
Description | CAS Common Chemistry is an open community resource for accessing chemical information. Nearly 500,000 chemical substances from CAS REGISTRY cover areas of community interest, including common and frequently regulated chemicals, and those relevant to high school and undergraduate chemistry classes. This chemical information, curated by our expert scientists, is provided in alignment with our mission as a division of the American Chemical Society. | |
Explanation | The data from CAS Common Chemistry is provided under a CC-BY-NC 4.0 license, unless otherwise stated. | |
Record name | Iodoacetonitrile | |
Source | ChemIDplus | |
URL | https://pubchem.ncbi.nlm.nih.gov/substance/?source=chemidplus&sourceid=0000624759 | |
Description | ChemIDplus is a free, web search system that provides access to the structure and nomenclature authority files used for the identification of chemical substances cited in National Library of Medicine (NLM) databases, including the TOXNET system. | |
Record name | 624-75-9 | |
Source | DTP/NCI | |
URL | https://dtp.cancer.gov/dtpstandard/servlet/dwindex?searchtype=NSC&outputformat=html&searchlist=81208 | |
Description | The NCI Development Therapeutics Program (DTP) provides services and resources to the academic and private-sector research communities worldwide to facilitate the discovery and development of new cancer therapeutic agents. | |
Explanation | Unless otherwise indicated, all text within NCI products is free of copyright and may be reused without our permission. Credit the National Cancer Institute as the source. | |
Record name | Acetonitrile, iodo- | |
Source | EPA DSSTox | |
URL | https://comptox.epa.gov/dashboard/DTXSID8073215 | |
Description | DSSTox provides a high quality public chemistry resource for supporting improved predictive toxicology. | |
Record name | Iodoacetonitrile | |
Source | European Chemicals Agency (ECHA) | |
URL | https://echa.europa.eu/substance-information/-/substanceinfo/100.009.873 | |
Description | The European Chemicals Agency (ECHA) is an agency of the European Union which is the driving force among regulatory authorities in implementing the EU's groundbreaking chemicals legislation for the benefit of human health and the environment as well as for innovation and competitiveness. | |
Explanation | Use of the information, documents and data from the ECHA website is subject to the terms and conditions of this Legal Notice, and subject to other binding limitations provided for under applicable law, the information, documents and data made available on the ECHA website may be reproduced, distributed and/or used, totally or in part, for non-commercial purposes provided that ECHA is acknowledged as the source: "Source: European Chemicals Agency, http://echa.europa.eu/". Such acknowledgement must be included in each copy of the material. ECHA permits and encourages organisations and individuals to create links to the ECHA website under the following cumulative conditions: Links can only be made to webpages that provide a link to the Legal Notice page. | |
Record name | Iodoacetonitrile | |
Source | FDA Global Substance Registration System (GSRS) | |
URL | https://gsrs.ncats.nih.gov/ginas/app/beta/substances/EXK5ZB5CF6 | |
Description | The FDA Global Substance Registration System (GSRS) enables the efficient and accurate exchange of information on what substances are in regulated products. Instead of relying on names, which vary across regulatory domains, countries, and regions, the GSRS knowledge base makes it possible for substances to be defined by standardized, scientific descriptions. | |
Explanation | Unless otherwise noted, the contents of the FDA website (www.fda.gov), both text and graphics, are not copyrighted. They are in the public domain and may be republished, reprinted and otherwise used freely by anyone without the need to obtain permission from FDA. Credit to the U.S. Food and Drug Administration as the source is appreciated but not required. | |
Retrosynthesis Analysis
AI-Powered Synthesis Planning: Our tool employs the Template_relevance Pistachio, Template_relevance Bkms_metabolic, Template_relevance Pistachio_ringbreaker, Template_relevance Reaxys, Template_relevance Reaxys_biocatalysis model, leveraging a vast database of chemical reactions to predict feasible synthetic routes.
One-Step Synthesis Focus: Specifically designed for one-step synthesis, it provides concise and direct routes for your target compounds, streamlining the synthesis process.
Accurate Predictions: Utilizing the extensive PISTACHIO, BKMS_METABOLIC, PISTACHIO_RINGBREAKER, REAXYS, REAXYS_BIOCATALYSIS database, our tool offers high-accuracy predictions, reflecting the latest in chemical research and data.
Strategy Settings
Precursor scoring | Relevance Heuristic |
---|---|
Min. plausibility | 0.01 |
Model | Template_relevance |
Template Set | Pistachio/Bkms_metabolic/Pistachio_ringbreaker/Reaxys/Reaxys_biocatalysis |
Top-N result to add to graph | 6 |
Feasible Synthetic Routes
Q1: What is the molecular formula and weight of iodoacetonitrile?
A1: this compound has a molecular formula of C2H2IN and a molecular weight of 166.94 g/mol.
Q2: Is there any spectroscopic data available for this compound?
A2: Yes, studies have characterized this compound using various spectroscopic techniques. For example, its microwave spectrum and quadrupole coupling have been investigated . Additionally, normal coordinate calculations have been performed using a seventeen-parameter valence force field to understand its vibrational modes .
Q3: How is this compound employed in organic synthesis?
A3: this compound serves as a versatile reagent in organic synthesis. It's commonly used as an alkylating agent, particularly for introducing cyanomethyl groups into molecules. For example, it's been successfully employed in the solid-phase synthesis of peptide C-terminal thioesters , the preparation of epoxysuccinate-based inhibitors of cysteine proteases , and the synthesis of substituted amidoundecanoic acids .
Q4: Can you elaborate on the use of this compound in controlled radical polymerization?
A4: Research indicates that this compound acts as an effective degenerative transfer agent in controlled radical polymerization. Studies have demonstrated its ability to control the polymerization of various monomers like methyl methacrylate, methyl acrylate, ethyl acrylate, vinyl acetate, and styrene, leading to polymers with controlled molecular weight and narrow molecular distribution . This control stems from the recirculating activation, fracture, and reformation of the carbon-iodo bond during polymerization.
Q5: How does this compound contribute to cyanomethylation reactions?
A5: this compound plays a crucial role in cyanomethylation reactions. It can be generated in situ from the iodination-decarboxylation of cyanoacetic acid and subsequently used for the cyanomethylation of amines and carboxylic acids, affording α-aminonitriles and cyanomethyl esters, respectively . This approach offers advantages like simple manipulation, inexpensive reagents, and a broad substrate scope.
Q6: Have any QSAR models been developed for this compound and related compounds?
A6: Yes, research has explored the quantitative structure-activity relationship (QSAR) of this compound and other haloacetonitriles, particularly in the context of their toxicity. Optimized QSAR models have been developed to predict cytotoxicity and genotoxicity based on their physicochemical parameters and toxicity metrics . These models are valuable for predicting the potential hazards of novel haloacetonitriles before conducting extensive biological evaluations.
Q7: What is known about the toxicity of this compound?
A7: this compound exhibits significant toxicity, particularly concerning its genotoxic properties. Studies have shown that this compound can induce DNA damage in mammalian cells . Furthermore, it can disrupt the normal cell cycle, leading to the accumulation of cells with abnormally high DNA content (hyperploidy) . This disruption can potentially contribute to adverse health outcomes, highlighting the importance of understanding and minimizing human exposure to this disinfection byproduct.
Q8: Is this compound found in drinking water, and if so, how does it form?
A8: Yes, this compound has been identified as an iodinated disinfection byproduct (I-DBP) in drinking water. While its formation is not as well-studied as some other DBPs, research suggests that it can form during chloramine disinfection of water containing iodide and organic matter . Interestingly, a recent study found that cooking pasta with iodized table salt in chloraminated tap water can also lead to the formation of this compound and other I-THMs .
Q9: What analytical methods are used to detect and quantify this compound?
A9: The analysis of this compound often involves gas chromatography coupled with mass spectrometry (GC-MS) techniques. Specifically, GC-MS/MS methods have been developed and optimized for the sensitive and reproducible measurement of this compound and other I-DBPs in complex matrices like cooked pasta and water .
Disclaimer and Information on In-Vitro Research Products
Please be aware that all articles and product information presented on BenchChem are intended solely for informational purposes. The products available for purchase on BenchChem are specifically designed for in-vitro studies, which are conducted outside of living organisms. In-vitro studies, derived from the Latin term "in glass," involve experiments performed in controlled laboratory settings using cells or tissues. It is important to note that these products are not categorized as medicines or drugs, and they have not received approval from the FDA for the prevention, treatment, or cure of any medical condition, ailment, or disease. We must emphasize that any form of bodily introduction of these products into humans or animals is strictly prohibited by law. It is essential to adhere to these guidelines to ensure compliance with legal and ethical standards in research and experimentation.