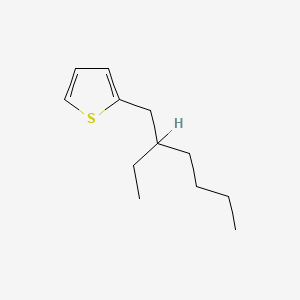
2-(2-Ethylhexyl)thiophene
Overview
Description
2-(2-Ethylhexyl)thiophene is a thiophene derivative featuring a branched 2-ethylhexyl substituent at the 2-position of the aromatic heterocycle. This compound is widely utilized in organic electronics, particularly as a building block for conjugated polymers in organic solar cells (OSCs). Its bulky alkyl chain enhances solubility in organic solvents and promotes optimal solid-state morphology in polymer blends, critical for charge transport in photovoltaic devices . For example, the polymer PBDTTT-EFT incorporates this compound units, achieving power conversion efficiencies (PCEs) exceeding 8% in bulk heterojunction solar cells . The compound’s synthesis often involves palladium-catalyzed cross-coupling reactions, as demonstrated in the preparation of derivatives like 2-(2-Ethylhexyl)-4-ethynylthiophene (96% yield) .
Preparation Methods
Synthetic Routes and Reaction Conditions: The synthesis of 2-(2-Ethylhexyl)thiophene typically involves the introduction of the 2-ethylhexyl group onto the thiophene ring. One common method is the Negishi coupling reaction, which involves the reaction of a thiophene derivative with a 2-ethylhexyl zincate reagent. This reaction is carried out under an inert atmosphere, typically using a palladium catalyst, and requires careful control of temperature and reaction time to achieve high yields .
Industrial Production Methods: In an industrial setting, the production of this compound may involve large-scale Negishi coupling reactions or other coupling methods such as Suzuki or Stille coupling. These methods are chosen based on factors such as cost, availability of reagents, and desired purity of the final product. The industrial process also includes steps for purification and quality control to ensure the compound meets the required specifications .
Chemical Reactions Analysis
Types of Reactions: 2-(2-Ethylhexyl)thiophene undergoes various chemical reactions, including:
Oxidation: The compound can be oxidized to form sulfoxides or sulfones, depending on the reaction conditions and oxidizing agents used.
Reduction: Reduction reactions can convert the thiophene ring to dihydrothiophene or tetrahydrothiophene derivatives.
Common Reagents and Conditions:
Oxidation: Common oxidizing agents include hydrogen peroxide, m-chloroperoxybenzoic acid, and potassium permanganate.
Reduction: Reducing agents such as lithium aluminum hydride or sodium borohydride are used under controlled conditions.
Substitution: Electrophilic reagents like bromine, chlorine, or nitronium ions are used in the presence of catalysts or under specific reaction conditions
Major Products:
Oxidation: Sulfoxides and sulfones.
Reduction: Dihydrothiophene and tetrahydrothiophene derivatives.
Substitution: Halogenated thiophenes, nitrothiophenes, and other substituted derivatives
Scientific Research Applications
Scientific Research Applications
- Organic Electronics : 2-(2-Ethylhexyl)thiophene serves as a key compound in organic electronics . Its properties contribute to enhancing device efficiency in applications like photovoltaics and OLEDs .
- Polymer Synthesis : It is used in the synthesis of poly[3-(2'-ethylhexyl)thiophene] (P3EHT), which can replace poly(3-hexylthiophene) (P3HT) in various applications . The use of P3EHT cores has demonstrated improved solubility .
- Biosensors : Thiophene-based trimers and polymers are used in biosensors for detecting various substances . For example, poly(acid-terthiophene)s have been used to detect H2O2 in urine samples . Also, they have been tested as electrochemical biosensors for evaluating the effect of endocrine disruptors .
- Sensing : Thiophene trimers have applications in sensing. For example, they have been used to detect brain-derived neurotrophic factor antibodies (BDNF) released from cancer cells, showing potential in drug screening and therapeutics .
- Pharmacological Activity : Terthiophene-methanol has been suggested as a potential candidate for further studies to evaluate its potential as an anti-cancer agent .
Case Studies
- Detection of H2O2: Silver nanoparticles attached to a BA-pTTP/carbon nanotubes composite were tested as a biosensor for the detection of H2O2 in urine samples, showing a fast response time (below 5s) and a LOD of 0.24 mM .
- Detection of Phthalate: Phthalate was analytically monitored with a microfluidic device coupled to a BA-pTTP electrochemical biosensor to evaluate the effect of endocrine disruptors. The biosensor exhibited high uptake ability towards soluble phthalate esters in aqueous media (LOD = 12.5 pM) by controlling the surface charge and hydrophobicity through assembling with a lipid and a cationic molecule attached to the BA-pTTP matrix .
- Detection of BDNF from Cancer Cells: COOH-biphenyl-functionalized trimers self-assembled on gold nanoparticles were used to covalently immobilize brain-derived neurotrophic factor antibodies (BDNF). The resulting microfluidic immunosensor detected the release of BDNF from various cancer cells by the effect of ethanol, K+, and nicotine, indicating its potential in drug screening and therapeutics .
Mechanism of Action
The mechanism of action of 2-(2-Ethylhexyl)thiophene in its various applications is largely dependent on its electronic structure and ability to interact with other molecules. In organic electronics, the compound’s conjugated system allows for efficient charge transport, making it suitable for use in semiconductors and other electronic devices. The presence of the 2-ethylhexyl group enhances solubility and processability, which is crucial for the fabrication of thin films and other materials .
Comparison with Similar Compounds
Structural Analogues with Alkyl Substituents
3-(2-Ethylhexyl)thiophene
- Structure : Alkyl chain at the 3-position of thiophene.
- Applications : Used in polymer synthesis for OSC active layers. Unlike 2-substituted isomers, 3-substitution may alter polymer backbone planarity, affecting charge mobility .
- Synthesis : Commercial availability (>97% purity) via Shanghai Zhenzhun Biotech .
2-Ethylthiophene
- Structure : Shorter ethyl chain at the 2-position.
- Properties: Lower molecular weight (126.23 g/mol) compared to 2-(2-ethylhexyl)thiophene (212.39 g/mol), leading to reduced solubility in nonpolar solvents .
- Applications: Primarily a precursor in pharmaceutical intermediates (e.g., 2-(2-thienyl)ethanol) .
2-(2-Butyloctyl)thiophene
- Structure : Longer alkyl chain (C12) at the 2-position.
- Performance : In polymers, longer alkyl chains improve film-forming properties but may reduce crystallinity, balancing solubility and charge transport .
Table 1. Key Properties of Alkyl-Substituted Thiophenes
Functionalized Thiophene Derivatives
2-(Phenylthio)thiophene (CAS 16718-12-0)
- Structure : Phenylthio group at the 2-position.
- Properties : Enhanced electron density due to sulfur and phenyl groups, making it suitable for organic semiconductors and sensors .
- Synthesis : Achieved via nucleophilic aromatic substitution or cross-coupling .
2-Acetylthiophene (CAS 88-15-3)
- Structure : Acetyl group at the 2-position.
- Applications : Used in flavoring agents and as a precursor for heterocyclic pharmaceuticals. Lacks the solubility advantages of alkylated thiophenes .
Table 2. Comparison of Functionalized Thiophenes
Performance in Organic Electronics
- This compound vs. 3-Hexylthiophene :
- P3HT (poly(3-hexylthiophene)) is a benchmark OSC material with PCEs ~4–5%. Incorporating this compound into copolymers (e.g., PBDTTT-EFT ) improves light absorption and PCEs (>8%) due to optimized bandgap and morphology .
- Bulkiness : The 2-ethylhexyl group reduces interchain π-π stacking compared to linear hexyl chains, balancing charge transport and solubility .
Biological Activity
2-(2-Ethylhexyl)thiophene is a thiophene derivative that has garnered attention for its potential biological activities, particularly in the context of antibacterial properties. This article synthesizes recent research findings, case studies, and data tables to provide a comprehensive overview of the biological activity associated with this compound.
This compound is characterized by its thiophene ring structure, which is known for its electronic properties and ability to participate in various chemical reactions. The presence of the ethylhexyl group enhances its solubility and bioavailability, making it a candidate for various applications in medicinal chemistry.
Antibacterial Activity
Recent studies have highlighted the antibacterial potential of this compound derivatives. A notable research effort focused on synthesizing various thiophene analogs, including 2-ethylhexyl 5-bromothiophene-2-carboxylate, which demonstrated significant antibacterial activity against multidrug-resistant strains such as XDR Salmonella Typhi.
Key Findings:
- Minimum Inhibitory Concentration (MIC) : The compound 2-ethylhexyl-5-(p-tolyl)thiophene-2-carboxylate exhibited an MIC value of 3.125 mg/mL against XDR Salmonella Typhi, outperforming traditional antibiotics like ciprofloxacin and ceftriaxone in efficacy .
- Structure-Activity Relationship (SAR) : The dual thiophene moieties in compound 4F were identified as critical for its enhanced antibacterial activity, suggesting that structural modifications can lead to improved pharmacological profiles .
Data Table: Antibacterial Efficacy of Thiophene Derivatives
Compound | MIC (mg/mL) | Target Bacteria | Comparison Antibiotic |
---|---|---|---|
2-Ethylhexyl-5-(p-tolyl)thiophene-2-carboxylate | 3.125 | XDR Salmonella Typhi | Ciprofloxacin |
2-Ethylhexyl-5-bromothiophene-2-carboxylate | 32 | E. coli | Ceftriaxone |
2,5-bis(4-chlorophenyl)-3-hexylthiophene | Not specified | S. aureus | Not specified |
Case Studies
- Molecular Docking Studies : Molecular docking studies were conducted to evaluate the binding affinities of synthesized thiophene derivatives against bacterial enzymes. The results indicated that certain modifications to the thiophene ring could enhance binding efficiency and antibacterial activity .
- In Vitro Testing : The agar well diffusion method was employed to assess the antibacterial activity of various thiophene derivatives. Results indicated a direct correlation between concentration and inhibition zone diameter, confirming the compounds' potential as effective antibacterial agents .
Toxicological Considerations
While exploring the biological activity of this compound, it is essential to consider its toxicological profile. Research has indicated potential acute and chronic toxicity risks associated with thiophene derivatives; however, specific data on this compound remains limited. Further studies are necessary to establish safety profiles and environmental impacts .
Q & A
Basic Research Questions
Q. What are the common synthetic routes for preparing 2-(2-Ethylhexyl)thiophene derivatives, and how are they optimized for reproducibility?
- Answer : The synthesis of this compound derivatives often employs Pd-catalyzed cross-coupling reactions (e.g., Stille or Suzuki couplings) to introduce functional groups or extend conjugation. For example, end-capping with 2-(tributylstannyl)thiophene ensures controlled polymer chain termination, critical for tuning molecular weight . Optimization involves rigorous control of reaction conditions (e.g., catalyst loading, solvent purity) and purification via column chromatography or Soxhlet extraction to remove metal residues. Reproducibility is validated by NMR spectroscopy and gel permeation chromatography (GPC) to confirm structural integrity and molecular weight distribution .
Q. What characterization techniques are essential for verifying the purity and structure of this compound-based polymers?
- Answer : Key techniques include:
- NMR spectroscopy (¹H/¹³C) to confirm substituent positions and branching.
- GPC to determine molecular weight and polydispersity.
- FTIR spectroscopy to identify functional groups (e.g., thiophene ring vibrations at ~690 cm⁻¹).
- Elemental analysis to validate empirical formulas.
- UV-Vis spectroscopy to assess optical bandgaps.
For example, in copolymer systems, discrepancies in NMR integration ratios may indicate incomplete monomer incorporation, requiring iterative synthesis adjustments .
Q. How should researchers handle and store this compound to ensure safety and stability?
- Answer : The compound should be stored in airtight, light-resistant containers under inert gas (e.g., argon) to prevent oxidation. Safety protocols include using fume hoods for synthesis, wearing nitrile gloves, and avoiding skin contact due to potential irritant properties . Waste disposal must follow institutional guidelines for halogenated organics. Stability tests (e.g., TGA/DSC) are recommended to assess thermal degradation thresholds .
Advanced Research Questions
Q. How can researchers resolve contradictions in reported charge mobility values for this compound-based polymers?
- Answer : Discrepancies in charge mobility (e.g., between field-effect transistor (FET) and space-charge-limited current (SCLC) measurements) often arise from differences in film morphology or testing conditions. To reconcile
- Standardize film preparation : Use identical spin-coating speeds and annealing protocols.
- Cross-validate techniques : Compare FET, SCLC, and time-resolved microwave conductivity (TRMC) results.
- Control environmental factors : Measure under inert conditions to exclude oxygen/water doping effects.
For instance, annealing temperature variations can alter polymer crystallinity, directly impacting mobility values .
Q. What strategies optimize the morphology of this compound-containing bulk heterojunction (BHJ) solar cells for enhanced efficiency?
- Answer : Morphology optimization involves:
- Solvent engineering : Using high-boiling-point additives (e.g., 1,8-diiodooctane) to slow crystallization, promoting phase-separated donor-acceptor networks.
- Thermal annealing : Post-deposition heating (e.g., 150°C for 10 min) to improve polymer chain alignment.
- Ternary blends : Incorporating a third component (e.g., PCBM derivatives) to balance charge transport.
For example, P3HT/PCBM systems achieve ~5% efficiency via solvent additive-driven phase separation, while newer polymers require tailored annealing protocols .
Q. How do steric effects from the 2-ethylhexyl substituent influence the electronic properties of thiophene-based polymers?
- Answer : The bulky 2-ethylhexyl group reduces interchain π-π stacking, lowering crystallinity but enhancing solubility for solution processing. This trade-off impacts charge transport:
- Reduced aggregation : Lowers hole mobility but minimizes trap states.
- Tunable bandgaps : Electron-withdrawing/donating substituents on the thiophene ring can offset steric effects.
Computational modeling (DFT) and grazing-incidence X-ray diffraction (GIXD) are critical for correlating substituent geometry with optoelectronic properties .
Q. Methodological Guidance
Q. How should researchers design experiments to analyze degradation mechanisms in this compound-based devices?
- Answer : Accelerated aging studies under controlled conditions (e.g., 85°C/85% humidity) combined with in situ characterization:
- FTIR/Raman spectroscopy to track chemical degradation (e.g., oxidation of thiophene rings).
- Impedance spectroscopy to monitor interfacial delamination.
- TOF-SIMS to map elemental diffusion at electrode interfaces.
For reproducibility, encapsulate devices with UV-curable resins and use inert-atmosphere gloveboxes for testing .
Q. What statistical methods are recommended for analyzing batch-to-batch variability in polymer synthesis?
- Answer : Employ multivariate analysis (e.g., PCA) to identify critical parameters (e.g., monomer purity, reaction time). Use ANOVA to compare molecular weight distributions across batches. For high-throughput screening, machine learning models can predict optimal synthesis conditions from historical data .
Q. Data Contradiction Analysis
Q. How to address conflicting reports on the environmental stability of this compound derivatives?
- Answer : Contradictions may stem from differing test environments (e.g., UV exposure vs. thermal stress). Standardize degradation protocols per ISO 4892-2 for UV testing and ASTM D638 for thermal aging. Cross-reference with computational degradation simulations (ReaxFF MD) to identify vulnerable molecular sites .
Properties
IUPAC Name |
2-(2-ethylhexyl)thiophene | |
---|---|---|
Source | PubChem | |
URL | https://pubchem.ncbi.nlm.nih.gov | |
Description | Data deposited in or computed by PubChem | |
InChI |
InChI=1S/C12H20S/c1-3-5-7-11(4-2)10-12-8-6-9-13-12/h6,8-9,11H,3-5,7,10H2,1-2H3 | |
Source | PubChem | |
URL | https://pubchem.ncbi.nlm.nih.gov | |
Description | Data deposited in or computed by PubChem | |
InChI Key |
JACCFQMSOHCQFN-UHFFFAOYSA-N | |
Source | PubChem | |
URL | https://pubchem.ncbi.nlm.nih.gov | |
Description | Data deposited in or computed by PubChem | |
Canonical SMILES |
CCCCC(CC)CC1=CC=CS1 | |
Source | PubChem | |
URL | https://pubchem.ncbi.nlm.nih.gov | |
Description | Data deposited in or computed by PubChem | |
Molecular Formula |
C12H20S | |
Source | PubChem | |
URL | https://pubchem.ncbi.nlm.nih.gov | |
Description | Data deposited in or computed by PubChem | |
Molecular Weight |
196.35 g/mol | |
Source | PubChem | |
URL | https://pubchem.ncbi.nlm.nih.gov | |
Description | Data deposited in or computed by PubChem | |
Retrosynthesis Analysis
AI-Powered Synthesis Planning: Our tool employs the Template_relevance Pistachio, Template_relevance Bkms_metabolic, Template_relevance Pistachio_ringbreaker, Template_relevance Reaxys, Template_relevance Reaxys_biocatalysis model, leveraging a vast database of chemical reactions to predict feasible synthetic routes.
One-Step Synthesis Focus: Specifically designed for one-step synthesis, it provides concise and direct routes for your target compounds, streamlining the synthesis process.
Accurate Predictions: Utilizing the extensive PISTACHIO, BKMS_METABOLIC, PISTACHIO_RINGBREAKER, REAXYS, REAXYS_BIOCATALYSIS database, our tool offers high-accuracy predictions, reflecting the latest in chemical research and data.
Strategy Settings
Precursor scoring | Relevance Heuristic |
---|---|
Min. plausibility | 0.01 |
Model | Template_relevance |
Template Set | Pistachio/Bkms_metabolic/Pistachio_ringbreaker/Reaxys/Reaxys_biocatalysis |
Top-N result to add to graph | 6 |
Feasible Synthetic Routes
Disclaimer and Information on In-Vitro Research Products
Please be aware that all articles and product information presented on BenchChem are intended solely for informational purposes. The products available for purchase on BenchChem are specifically designed for in-vitro studies, which are conducted outside of living organisms. In-vitro studies, derived from the Latin term "in glass," involve experiments performed in controlled laboratory settings using cells or tissues. It is important to note that these products are not categorized as medicines or drugs, and they have not received approval from the FDA for the prevention, treatment, or cure of any medical condition, ailment, or disease. We must emphasize that any form of bodily introduction of these products into humans or animals is strictly prohibited by law. It is essential to adhere to these guidelines to ensure compliance with legal and ethical standards in research and experimentation.