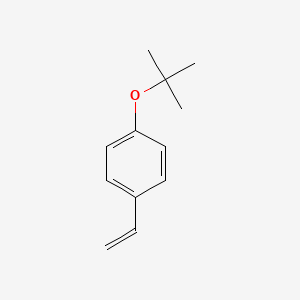
4-tert-Butoxystyrene
Overview
Description
4-tert-butoxystyrene is a pendant functionalized styrene. Structurally, it is similar to PMOS, both carrying an alkoxyl p-substituent, whose electron-donating and resonance effects allow them to be polymerized by cationic, anionic, and radical mechanisms .
Synthesis Analysis
Cationic polymerization of 4-tert-butoxystyrene initiated by hydrogen iodide/zinc iodide (HI/ZnI2) led to well-defined living polymers at temperatures up to +25°C in either toluene or methylene chloride . Other research also mentions the synthesis of block copolymers using this compound .Molecular Structure Analysis
The molecular formula of 4-tert-Butoxystyrene is C12H16O. It has a molecular weight of 176.25 g/mol. The InChIKey is GRFNSWBVXHLTCI-UHFFFAOYSA-N .Chemical Reactions Analysis
The cationic polymerization of 4-tert-butoxystyrene has been reported in the literature . Another study mentions the use of RAFT-mediated polymerization-induced self-assembly (RAFT-PISA) with this compound .Physical And Chemical Properties Analysis
4-tert-Butoxystyrene is a liquid at 20°C. It has a specific gravity of 0.94 and a refractive index of 1.52. It has a topological polar surface area of 9.2 Ų .Scientific Research Applications
Hydrolysis to Poly(4-hydroxystyrene)
Poly(4-tert-butoxystyrene) can be converted into poly(4-hydroxystyrene) through hydrolysis using strong acid. This process is significant for investigating the molecular characteristics of the polymer upon reaction .
Radical Polymerization
TEMPO, a chemical compound, has been reported to induce the formation of initiator radicals for autopolymerization of styrene, which includes derivatives like 4-tert-Butoxystyrene. This application is crucial for creating polymers with narrow polydispersity .
Micelle Formation
Polystyrene-block-poly(4-tert-butoxystyrene) has been observed to form micelles in a solution, which can be analyzed using dynamic light-scattering (DLS). The formation of micelles is essential for applications in drug delivery systems and nanotechnology .
Block Copolymer Synthesis
Block copolymers of poly(4-tert-butoxystyrene) have been prepared by sequential addition of monomers using specific initiators. This synthesis is vital for creating materials with unique properties for advanced applications .
Distillation and Storage
The compound is often dried over calcium hydride and distilled under reduced pressure before being stored under nitrogen to preserve its quality. Proper distillation and storage are crucial for ensuring the compound’s effectiveness in subsequent reactions .
Safety And Hazards
Future Directions
The future directions of 4-tert-Butoxystyrene research could involve its use in the synthesis of block copolymers and the exploration of RAFT-mediated polymerization-induced self-assembly (RAFT-PISA). This method combines polymerization and self-assembly in a single step, setting it apart from conventional solution self-assembly processes .
properties
IUPAC Name |
1-ethenyl-4-[(2-methylpropan-2-yl)oxy]benzene | |
---|---|---|
Source | PubChem | |
URL | https://pubchem.ncbi.nlm.nih.gov | |
Description | Data deposited in or computed by PubChem | |
InChI |
InChI=1S/C12H16O/c1-5-10-6-8-11(9-7-10)13-12(2,3)4/h5-9H,1H2,2-4H3 | |
Source | PubChem | |
URL | https://pubchem.ncbi.nlm.nih.gov | |
Description | Data deposited in or computed by PubChem | |
InChI Key |
GRFNSWBVXHLTCI-UHFFFAOYSA-N | |
Source | PubChem | |
URL | https://pubchem.ncbi.nlm.nih.gov | |
Description | Data deposited in or computed by PubChem | |
Canonical SMILES |
CC(C)(C)OC1=CC=C(C=C1)C=C | |
Source | PubChem | |
URL | https://pubchem.ncbi.nlm.nih.gov | |
Description | Data deposited in or computed by PubChem | |
Molecular Formula |
C12H16O | |
Source | PubChem | |
URL | https://pubchem.ncbi.nlm.nih.gov | |
Description | Data deposited in or computed by PubChem | |
Related CAS |
95418-60-3 | |
Record name | Benzene, 1-(1,1-dimethylethoxy)-4-ethenyl-, homopolymer | |
Source | CAS Common Chemistry | |
URL | https://commonchemistry.cas.org/detail?cas_rn=95418-60-3 | |
Description | CAS Common Chemistry is an open community resource for accessing chemical information. Nearly 500,000 chemical substances from CAS REGISTRY cover areas of community interest, including common and frequently regulated chemicals, and those relevant to high school and undergraduate chemistry classes. This chemical information, curated by our expert scientists, is provided in alignment with our mission as a division of the American Chemical Society. | |
Explanation | The data from CAS Common Chemistry is provided under a CC-BY-NC 4.0 license, unless otherwise stated. | |
DSSTOX Substance ID |
DTXSID30341978 | |
Record name | 4-tert-Butoxystyrene | |
Source | EPA DSSTox | |
URL | https://comptox.epa.gov/dashboard/DTXSID30341978 | |
Description | DSSTox provides a high quality public chemistry resource for supporting improved predictive toxicology. | |
Molecular Weight |
176.25 g/mol | |
Source | PubChem | |
URL | https://pubchem.ncbi.nlm.nih.gov | |
Description | Data deposited in or computed by PubChem | |
Product Name |
4-tert-Butoxystyrene | |
CAS RN |
95418-58-9 | |
Record name | 1-(1,1-Dimethylethoxy)-4-ethenylbenzene | |
Source | CAS Common Chemistry | |
URL | https://commonchemistry.cas.org/detail?cas_rn=95418-58-9 | |
Description | CAS Common Chemistry is an open community resource for accessing chemical information. Nearly 500,000 chemical substances from CAS REGISTRY cover areas of community interest, including common and frequently regulated chemicals, and those relevant to high school and undergraduate chemistry classes. This chemical information, curated by our expert scientists, is provided in alignment with our mission as a division of the American Chemical Society. | |
Explanation | The data from CAS Common Chemistry is provided under a CC-BY-NC 4.0 license, unless otherwise stated. | |
Record name | 4-tert-Butoxystyrene | |
Source | EPA DSSTox | |
URL | https://comptox.epa.gov/dashboard/DTXSID30341978 | |
Description | DSSTox provides a high quality public chemistry resource for supporting improved predictive toxicology. | |
Synthesis routes and methods
Procedure details
Retrosynthesis Analysis
AI-Powered Synthesis Planning: Our tool employs the Template_relevance Pistachio, Template_relevance Bkms_metabolic, Template_relevance Pistachio_ringbreaker, Template_relevance Reaxys, Template_relevance Reaxys_biocatalysis model, leveraging a vast database of chemical reactions to predict feasible synthetic routes.
One-Step Synthesis Focus: Specifically designed for one-step synthesis, it provides concise and direct routes for your target compounds, streamlining the synthesis process.
Accurate Predictions: Utilizing the extensive PISTACHIO, BKMS_METABOLIC, PISTACHIO_RINGBREAKER, REAXYS, REAXYS_BIOCATALYSIS database, our tool offers high-accuracy predictions, reflecting the latest in chemical research and data.
Strategy Settings
Precursor scoring | Relevance Heuristic |
---|---|
Min. plausibility | 0.01 |
Model | Template_relevance |
Template Set | Pistachio/Bkms_metabolic/Pistachio_ringbreaker/Reaxys/Reaxys_biocatalysis |
Top-N result to add to graph | 6 |
Feasible Synthetic Routes
Disclaimer and Information on In-Vitro Research Products
Please be aware that all articles and product information presented on BenchChem are intended solely for informational purposes. The products available for purchase on BenchChem are specifically designed for in-vitro studies, which are conducted outside of living organisms. In-vitro studies, derived from the Latin term "in glass," involve experiments performed in controlled laboratory settings using cells or tissues. It is important to note that these products are not categorized as medicines or drugs, and they have not received approval from the FDA for the prevention, treatment, or cure of any medical condition, ailment, or disease. We must emphasize that any form of bodily introduction of these products into humans or animals is strictly prohibited by law. It is essential to adhere to these guidelines to ensure compliance with legal and ethical standards in research and experimentation.