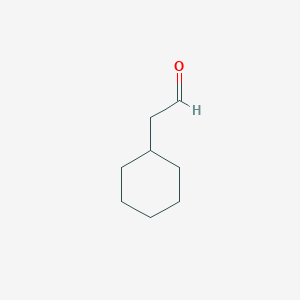
2-Cyclohexylacetaldehyde
Overview
Description
2-Cyclohexylacetaldehyde is an organic compound with the molecular formula C8H14O . It is a colorless liquid with a distinctive green-floral odor. This compound is primarily used in the fragrance industry due to its pleasant scent, which makes it suitable for various household products .
Preparation Methods
Synthetic Routes and Reaction Conditions: 2-Cyclohexylacetaldehyde is synthesized through the condensation of butadiene with crotonaldehyde, followed by hydrogenation . The reaction conditions typically involve:
Condensation: This step is carried out under controlled temperatures and pressures to ensure the efficient formation of the intermediate product.
Hydrogenation: The intermediate product is then hydrogenated using a suitable catalyst, such as palladium or nickel, under high pressure and temperature to yield cyclohexylacetaldehyde.
Industrial Production Methods: In industrial settings, the production of cyclohexylacetaldehyde follows the same synthetic route but on a larger scale. The process involves:
Large-scale reactors: These are used to handle the high volumes of reactants and products.
Continuous monitoring: This ensures the reaction conditions are maintained within the desired range to optimize yield and purity.
Chemical Reactions Analysis
Types of Reactions: 2-Cyclohexylacetaldehyde undergoes several types of chemical reactions, including:
Oxidation: It can be oxidized to form cyclohexylacetic acid.
Reduction: It can be reduced to cyclohexylethanol.
Substitution: It can undergo nucleophilic substitution reactions to form various derivatives.
Common Reagents and Conditions:
Oxidation: Common oxidizing agents include potassium permanganate (KMnO4) and chromium trioxide (CrO3).
Reduction: Reducing agents such as sodium borohydride (NaBH4) or lithium aluminum hydride (LiAlH4) are typically used.
Substitution: Nucleophiles like amines or alcohols can be used under acidic or basic conditions.
Major Products:
Oxidation: Cyclohexylacetic acid.
Reduction: Cyclohexylethanol.
Substitution: Various cyclohexyl derivatives depending on the nucleophile used.
Scientific Research Applications
2-Cyclohexylacetaldehyde has several applications in scientific research, including:
Chemistry: It is used as an intermediate in the synthesis of various organic compounds.
Biology: It serves as a model compound in studies involving aldehyde reactivity and metabolism.
Medicine: Research is ongoing to explore its potential therapeutic applications, particularly in the development of new drugs.
Industry: Apart from its use in fragrances, it is also used in the production of specialty chemicals and as a flavoring agent .
Mechanism of Action
The mechanism of action of cyclohexylacetaldehyde involves its interaction with various molecular targets and pathways. As an aldehyde, it can form Schiff bases with amines, which are key intermediates in many biochemical reactions. Additionally, its reactivity with nucleophiles makes it a versatile compound in organic synthesis .
Comparison with Similar Compounds
2-Cyclohexylacetaldehyde can be compared with other similar compounds such as:
Cyclohexanecarboxaldehyde: Similar in structure but differs in the position of the aldehyde group.
Cyclohexylmethyl ketone: Contains a ketone group instead of an aldehyde.
Cyclohexylacetic acid: The oxidized form of cyclohexylacetaldehyde.
Uniqueness: this compound’s unique combination of a cyclohexyl ring and an aldehyde group makes it particularly valuable in fragrance applications and as an intermediate in organic synthesis .
Properties
IUPAC Name |
2-cyclohexylacetaldehyde | |
---|---|---|
Source | PubChem | |
URL | https://pubchem.ncbi.nlm.nih.gov | |
Description | Data deposited in or computed by PubChem | |
InChI |
InChI=1S/C8H14O/c9-7-6-8-4-2-1-3-5-8/h7-8H,1-6H2 | |
Source | PubChem | |
URL | https://pubchem.ncbi.nlm.nih.gov | |
Description | Data deposited in or computed by PubChem | |
InChI Key |
JJMDTERTPNYIGZ-UHFFFAOYSA-N | |
Source | PubChem | |
URL | https://pubchem.ncbi.nlm.nih.gov | |
Description | Data deposited in or computed by PubChem | |
Canonical SMILES |
C1CCC(CC1)CC=O | |
Source | PubChem | |
URL | https://pubchem.ncbi.nlm.nih.gov | |
Description | Data deposited in or computed by PubChem | |
Molecular Formula |
C8H14O | |
Source | PubChem | |
URL | https://pubchem.ncbi.nlm.nih.gov | |
Description | Data deposited in or computed by PubChem | |
DSSTOX Substance ID |
DTXSID40311703 | |
Record name | 2-cyclohexylacetaldehyde | |
Source | EPA DSSTox | |
URL | https://comptox.epa.gov/dashboard/DTXSID40311703 | |
Description | DSSTox provides a high quality public chemistry resource for supporting improved predictive toxicology. | |
Molecular Weight |
126.20 g/mol | |
Source | PubChem | |
URL | https://pubchem.ncbi.nlm.nih.gov | |
Description | Data deposited in or computed by PubChem | |
CAS No. |
5664-21-1 | |
Record name | 2-Cyclohexylacetaldehyde | |
Source | ChemIDplus | |
URL | https://pubchem.ncbi.nlm.nih.gov/substance/?source=chemidplus&sourceid=0005664211 | |
Description | ChemIDplus is a free, web search system that provides access to the structure and nomenclature authority files used for the identification of chemical substances cited in National Library of Medicine (NLM) databases, including the TOXNET system. | |
Record name | Cyclohexaneacetaldehyde | |
Source | DTP/NCI | |
URL | https://dtp.cancer.gov/dtpstandard/servlet/dwindex?searchtype=NSC&outputformat=html&searchlist=244934 | |
Description | The NCI Development Therapeutics Program (DTP) provides services and resources to the academic and private-sector research communities worldwide to facilitate the discovery and development of new cancer therapeutic agents. | |
Explanation | Unless otherwise indicated, all text within NCI products is free of copyright and may be reused without our permission. Credit the National Cancer Institute as the source. | |
Record name | 2-cyclohexylacetaldehyde | |
Source | EPA DSSTox | |
URL | https://comptox.epa.gov/dashboard/DTXSID40311703 | |
Description | DSSTox provides a high quality public chemistry resource for supporting improved predictive toxicology. | |
Record name | 2-cyclohexylacetaldehyde | |
Source | European Chemicals Agency (ECHA) | |
URL | https://echa.europa.eu/information-on-chemicals | |
Description | The European Chemicals Agency (ECHA) is an agency of the European Union which is the driving force among regulatory authorities in implementing the EU's groundbreaking chemicals legislation for the benefit of human health and the environment as well as for innovation and competitiveness. | |
Explanation | Use of the information, documents and data from the ECHA website is subject to the terms and conditions of this Legal Notice, and subject to other binding limitations provided for under applicable law, the information, documents and data made available on the ECHA website may be reproduced, distributed and/or used, totally or in part, for non-commercial purposes provided that ECHA is acknowledged as the source: "Source: European Chemicals Agency, http://echa.europa.eu/". Such acknowledgement must be included in each copy of the material. ECHA permits and encourages organisations and individuals to create links to the ECHA website under the following cumulative conditions: Links can only be made to webpages that provide a link to the Legal Notice page. | |
Record name | 2-Cyclohexylacetaldehyde | |
Source | FDA Global Substance Registration System (GSRS) | |
URL | https://gsrs.ncats.nih.gov/ginas/app/beta/substances/YYW2A23ZV3 | |
Description | The FDA Global Substance Registration System (GSRS) enables the efficient and accurate exchange of information on what substances are in regulated products. Instead of relying on names, which vary across regulatory domains, countries, and regions, the GSRS knowledge base makes it possible for substances to be defined by standardized, scientific descriptions. | |
Explanation | Unless otherwise noted, the contents of the FDA website (www.fda.gov), both text and graphics, are not copyrighted. They are in the public domain and may be republished, reprinted and otherwise used freely by anyone without the need to obtain permission from FDA. Credit to the U.S. Food and Drug Administration as the source is appreciated but not required. | |
Synthesis routes and methods I
Procedure details
Synthesis routes and methods II
Procedure details
Synthesis routes and methods III
Procedure details
Synthesis routes and methods IV
Procedure details
Retrosynthesis Analysis
AI-Powered Synthesis Planning: Our tool employs the Template_relevance Pistachio, Template_relevance Bkms_metabolic, Template_relevance Pistachio_ringbreaker, Template_relevance Reaxys, Template_relevance Reaxys_biocatalysis model, leveraging a vast database of chemical reactions to predict feasible synthetic routes.
One-Step Synthesis Focus: Specifically designed for one-step synthesis, it provides concise and direct routes for your target compounds, streamlining the synthesis process.
Accurate Predictions: Utilizing the extensive PISTACHIO, BKMS_METABOLIC, PISTACHIO_RINGBREAKER, REAXYS, REAXYS_BIOCATALYSIS database, our tool offers high-accuracy predictions, reflecting the latest in chemical research and data.
Strategy Settings
Precursor scoring | Relevance Heuristic |
---|---|
Min. plausibility | 0.01 |
Model | Template_relevance |
Template Set | Pistachio/Bkms_metabolic/Pistachio_ringbreaker/Reaxys/Reaxys_biocatalysis |
Top-N result to add to graph | 6 |
Feasible Synthetic Routes
Disclaimer and Information on In-Vitro Research Products
Please be aware that all articles and product information presented on BenchChem are intended solely for informational purposes. The products available for purchase on BenchChem are specifically designed for in-vitro studies, which are conducted outside of living organisms. In-vitro studies, derived from the Latin term "in glass," involve experiments performed in controlled laboratory settings using cells or tissues. It is important to note that these products are not categorized as medicines or drugs, and they have not received approval from the FDA for the prevention, treatment, or cure of any medical condition, ailment, or disease. We must emphasize that any form of bodily introduction of these products into humans or animals is strictly prohibited by law. It is essential to adhere to these guidelines to ensure compliance with legal and ethical standards in research and experimentation.