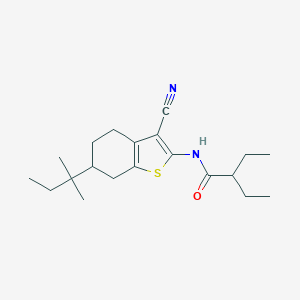
Glucagon Receptor Antagonist I
Overview
Description
The human glucagon receptor antagonist is a compound that inhibits the action of glucagon by blocking its receptor, the glucagon receptor. Glucagon is a hormone produced by the pancreas that raises blood glucose levels by promoting gluconeogenesis and glycogenolysis in the liver. Antagonists of the glucagon receptor are being studied for their potential to treat diabetes by lowering blood glucose levels .
Scientific Research Applications
Human glucagon receptor antagonists have a wide range of scientific research applications:
Chemistry: Used as tools to study receptor-ligand interactions and to develop new synthetic methodologies.
Biology: Employed in research to understand the role of glucagon in glucose metabolism and its regulation.
Medicine: Investigated for their potential to treat diabetes by lowering blood glucose levels. .
Industry: Used in the development of new therapeutic agents and in the production of research chemicals.
Mechanism of Action
Target of Action
The primary target of the Glucagon Receptor Antagonist I, also known as hGCGR Antagonist, is the glucagon receptor (GCGR) . This receptor is a G-protein-coupled receptor (GPCR) mainly detected in islet β cells and liver cells . Glucagon, a hormone secreted by pancreatic α cells, binds to this receptor and promotes hepatic glycogen breakdown and increases blood glucose levels .
Mode of Action
The hGCGR Antagonist inhibits the binding of glucagon to the human glucagon receptor . This inhibition results in an increased half-maximal effect for glucagon stimulation of adenylyl cyclase . The antagonist also blocks glucagon-mediated glycogenolysis in primary human hepatocytes . This means that the antagonist prevents the breakdown of glycogen into glucose in the liver, thereby reducing blood glucose levels .
Biochemical Pathways
The hGCGR Antagonist affects several biochemical pathways. By blocking the glucagon receptor, it inhibits the cAMP–PKA pathway, which is involved in the breakdown of glycogen . It also impacts lipid metabolism. In hepatocytes, glucagon may bring about an energy-depleted state (increasing the AMP/ATP ratio) sufficient to activate AMP-activated kinase . This kinase phosphorylates acetyl-CoA carboxylase and p38 mitogen-activated protein kinase, leading to transcriptional activation of peroxisome proliferator-activated receptor-α (PPARα) . PPARα stimulates the transcription of genes involved in beta-oxidation .
Pharmacokinetics
For instance, RN909, a monoclonal antibody antagonist of the glucagon receptor, was well-tolerated after single and multiple doses in type 2 diabetes subjects .
Result of Action
The hGCGR Antagonist’s action results in molecular and cellular effects that contribute to the reduction of blood glucose levels . By blocking the glucagon receptor, it inhibits the breakdown of glycogen into glucose in the liver . This leads to a reduction in blood glucose levels . Additionally, it has been suggested that glucagon receptor antagonism can relieve hyperglycemia in animals and humans .
Action Environment
The action of the hGCGR Antagonist can be influenced by various environmental factors. For instance, the presence of other hormones, such as insulin, can impact its efficacy . Furthermore, the antagonist’s action can be affected by the metabolic state of the individual, such as whether they are in a fasting or postprandial state
Future Directions
Emerging data support an essential role of glucagon for lipid metabolism . The potential of emerging GLP-1RAs and GLP-1/glucagon receptor co-agonists in the treatment of NAFLD is being explored . The studies provide important new information that further illuminates pathways and strategies to optimize the use of new GLP-1R agonists for the treatment of human subjects with diabetes and/or obesity .
Biochemical Analysis
Biochemical Properties
Glucagon Receptor Antagonist I interacts with the glucagon receptor, which is a G-protein-coupled receptor . When glucagon binds to GCGR, it promotes liver glycogen breakdown and increases blood glucose levels . This compound blocks this interaction, thereby potentially reducing blood glucose levels .
Cellular Effects
This compound has been shown to have significant effects on various types of cells and cellular processes. For instance, it has been reported to improve glucose control and upregulate plasma insulin level . It also influences cell function by impacting cell signaling pathways, gene expression, and cellular metabolism .
Molecular Mechanism
The molecular mechanism of this compound involves its binding to the glucagon receptor, thereby preventing the activation of the receptor . This prevents the full coupling of the G protein and the activation of the receptor , which in turn inhibits the downstream signaling pathways that lead to increased blood glucose levels .
Temporal Effects in Laboratory Settings
In laboratory settings, this compound has been observed to have durable fasting plasma glucose (FPG)-lowering effects at day 29 and day 85 after single and multiple doses, respectively . It also reduces HbA1c levels .
Dosage Effects in Animal Models
In animal models, the effects of this compound vary with different dosages . For instance, it has been reported to ameliorate the development of nonalcoholic fatty liver disease (NAFLD) by lowering body weight, improving liver and lipid profiles, and reducing the levels of the fibrosis marker PRO-C4 .
Metabolic Pathways
This compound is involved in the regulation of lipid and cholic acid metabolism . It interacts with enzymes and cofactors in these metabolic pathways, influencing metabolic flux or metabolite levels .
Preparation Methods
Synthetic Routes and Reaction Conditions
The synthesis of human glucagon receptor antagonists involves multiple steps, including the formation of key intermediates and the final coupling reactions. For example, one synthetic route involves the preparation of N-[3-cyano-6-(1,1-dimethylpropyl)-4,5,6,7-tetrahydro-1-benzothien-2-yl]-2-ethylbutanamide, a potent glucagon receptor antagonist . The reaction conditions typically involve the use of organic solvents, catalysts, and controlled temperatures to ensure high yields and purity.
Industrial Production Methods
Industrial production of human glucagon receptor antagonists follows similar synthetic routes but on a larger scale. This involves optimizing reaction conditions for scalability, including the use of large reactors, efficient purification methods, and stringent quality control measures to ensure consistency and safety of the final product .
Chemical Reactions Analysis
Types of Reactions
Human glucagon receptor antagonists undergo various chemical reactions, including:
Oxidation: Involves the addition of oxygen or the removal of hydrogen.
Reduction: Involves the addition of hydrogen or the removal of oxygen.
Substitution: Involves the replacement of one functional group with another.
Common Reagents and Conditions
Common reagents used in these reactions include oxidizing agents like potassium permanganate, reducing agents like lithium aluminum hydride, and various nucleophiles for substitution reactions. Reaction conditions often involve specific temperatures, pressures, and pH levels to optimize the reaction rates and yields .
Major Products
The major products formed from these reactions are typically the desired glucagon receptor antagonists, along with by-products that are removed during purification. The final product is characterized by its ability to inhibit the glucagon receptor effectively .
Comparison with Similar Compounds
Human glucagon receptor antagonists can be compared with other similar compounds, such as:
Dipeptidyl peptidase-4 inhibitors: These compounds also lower blood glucose levels but through a different mechanism involving the inhibition of the enzyme dipeptidyl peptidase-4.
Glucagon-like peptide-1 receptor agonists: These compounds mimic the action of glucagon-like peptide-1 to enhance insulin secretion and lower blood glucose levels.
The uniqueness of human glucagon receptor antagonists lies in their specific targeting of the glucagon receptor, which directly inhibits the action of glucagon and provides a novel approach to diabetes treatment .
Properties
IUPAC Name |
N-[3-cyano-6-(2-methylbutan-2-yl)-4,5,6,7-tetrahydro-1-benzothiophen-2-yl]-2-ethylbutanamide | |
---|---|---|
Source | PubChem | |
URL | https://pubchem.ncbi.nlm.nih.gov | |
Description | Data deposited in or computed by PubChem | |
InChI |
InChI=1S/C20H30N2OS/c1-6-13(7-2)18(23)22-19-16(12-21)15-10-9-14(11-17(15)24-19)20(4,5)8-3/h13-14H,6-11H2,1-5H3,(H,22,23) | |
Source | PubChem | |
URL | https://pubchem.ncbi.nlm.nih.gov | |
Description | Data deposited in or computed by PubChem | |
InChI Key |
SWIBDWBSJSJQHL-UHFFFAOYSA-N | |
Source | PubChem | |
URL | https://pubchem.ncbi.nlm.nih.gov | |
Description | Data deposited in or computed by PubChem | |
Canonical SMILES |
CCC(CC)C(=O)NC1=C(C2=C(S1)CC(CC2)C(C)(C)CC)C#N | |
Source | PubChem | |
URL | https://pubchem.ncbi.nlm.nih.gov | |
Description | Data deposited in or computed by PubChem | |
Molecular Formula |
C20H30N2OS | |
Source | PubChem | |
URL | https://pubchem.ncbi.nlm.nih.gov | |
Description | Data deposited in or computed by PubChem | |
DSSTOX Substance ID |
DTXSID70347212 | |
Record name | Glucagon Receptor Antagonist I | |
Source | EPA DSSTox | |
URL | https://comptox.epa.gov/dashboard/DTXSID70347212 | |
Description | DSSTox provides a high quality public chemistry resource for supporting improved predictive toxicology. | |
Molecular Weight |
346.5 g/mol | |
Source | PubChem | |
URL | https://pubchem.ncbi.nlm.nih.gov | |
Description | Data deposited in or computed by PubChem | |
CAS No. |
438618-32-7 | |
Record name | Glucagon Receptor Antagonist I | |
Source | EPA DSSTox | |
URL | https://comptox.epa.gov/dashboard/DTXSID70347212 | |
Description | DSSTox provides a high quality public chemistry resource for supporting improved predictive toxicology. | |
Retrosynthesis Analysis
AI-Powered Synthesis Planning: Our tool employs the Template_relevance Pistachio, Template_relevance Bkms_metabolic, Template_relevance Pistachio_ringbreaker, Template_relevance Reaxys, Template_relevance Reaxys_biocatalysis model, leveraging a vast database of chemical reactions to predict feasible synthetic routes.
One-Step Synthesis Focus: Specifically designed for one-step synthesis, it provides concise and direct routes for your target compounds, streamlining the synthesis process.
Accurate Predictions: Utilizing the extensive PISTACHIO, BKMS_METABOLIC, PISTACHIO_RINGBREAKER, REAXYS, REAXYS_BIOCATALYSIS database, our tool offers high-accuracy predictions, reflecting the latest in chemical research and data.
Strategy Settings
Precursor scoring | Relevance Heuristic |
---|---|
Min. plausibility | 0.01 |
Model | Template_relevance |
Template Set | Pistachio/Bkms_metabolic/Pistachio_ringbreaker/Reaxys/Reaxys_biocatalysis |
Top-N result to add to graph | 6 |
Feasible Synthetic Routes
Disclaimer and Information on In-Vitro Research Products
Please be aware that all articles and product information presented on BenchChem are intended solely for informational purposes. The products available for purchase on BenchChem are specifically designed for in-vitro studies, which are conducted outside of living organisms. In-vitro studies, derived from the Latin term "in glass," involve experiments performed in controlled laboratory settings using cells or tissues. It is important to note that these products are not categorized as medicines or drugs, and they have not received approval from the FDA for the prevention, treatment, or cure of any medical condition, ailment, or disease. We must emphasize that any form of bodily introduction of these products into humans or animals is strictly prohibited by law. It is essential to adhere to these guidelines to ensure compliance with legal and ethical standards in research and experimentation.