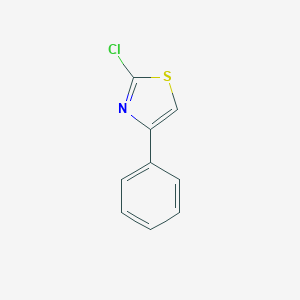
2-Chloro-4-phenylthiazole
Overview
Description
2-Chloro-4-phenylthiazole is a heterocyclic compound that belongs to the thiazole family. Thiazoles are five-membered aromatic rings containing both sulfur and nitrogen atoms. The presence of a chlorine atom at the second position and a phenyl group at the fourth position makes this compound a unique and versatile compound. It is known for its significant biological and chemical properties, making it a valuable compound in various fields of research and industry .
Mechanism of Action
Target of Action
This compound belongs to the thiazole class of compounds, which are known to exhibit a wide range of biological activities . .
Mode of Action
Thiazoles, in general, are known to interact with various biological targets through different mechanisms . The specific interactions of 2-Chloro-4-phenylthiazole with its targets, and the resulting changes, are subjects of ongoing research.
Biochemical Pathways
Thiazole derivatives have been reported to influence a variety of biochemical pathways, leading to diverse biological effects . .
Result of Action
Thiazole derivatives have been associated with a range of biological activities, including antimicrobial, antifungal, anti-inflammatory, and antioxidant effects . .
Biochemical Analysis
Biochemical Properties
2-Chloro-4-phenylthiazole plays a significant role in biochemical reactions due to its ability to interact with various enzymes, proteins, and other biomolecules. It has been found to exhibit antibacterial, antifungal, anti-inflammatory, and antitumor activities . The compound interacts with enzymes such as acetylcholinesterase, inhibiting its activity and thus affecting neurotransmission . Additionally, this compound has been shown to bind to proteins involved in oxidative stress response, thereby modulating their activity and contributing to its antioxidant properties .
Cellular Effects
This compound influences various cellular processes, including cell signaling pathways, gene expression, and cellular metabolism. It has been observed to induce apoptosis in cancer cells by activating caspase enzymes and promoting the release of cytochrome c from mitochondria . The compound also affects cell signaling pathways such as the MAPK/ERK pathway, leading to altered gene expression and inhibition of cell proliferation . Furthermore, this compound has been reported to modulate cellular metabolism by inhibiting key metabolic enzymes, resulting in reduced ATP production and increased oxidative stress .
Molecular Mechanism
The molecular mechanism of this compound involves its interaction with various biomolecules at the molecular level. The compound binds to the active sites of enzymes, inhibiting their activity and leading to downstream effects on cellular processes . For example, its binding to acetylcholinesterase results in the accumulation of acetylcholine in synaptic clefts, affecting neurotransmission . Additionally, this compound has been shown to interact with DNA, causing changes in gene expression and promoting apoptosis in cancer cells .
Temporal Effects in Laboratory Settings
In laboratory settings, the effects of this compound have been observed to change over time. The compound is relatively stable under standard laboratory conditions, but it can degrade when exposed to light and high temperatures . Long-term studies have shown that this compound can have sustained effects on cellular function, including prolonged inhibition of enzyme activity and persistent changes in gene expression . These effects are particularly evident in in vitro studies, where the compound’s stability and degradation can be closely monitored .
Dosage Effects in Animal Models
The effects of this compound vary with different dosages in animal models. At low doses, the compound exhibits therapeutic effects such as anti-inflammatory and antitumor activities . At high doses, it can cause toxic effects, including hepatotoxicity and nephrotoxicity . Threshold effects have been observed, where the compound’s beneficial effects are maximized at specific dosages, beyond which adverse effects become more pronounced . These findings highlight the importance of dosage optimization in the therapeutic use of this compound .
Metabolic Pathways
This compound is involved in various metabolic pathways, interacting with enzymes and cofactors that regulate its metabolism. The compound is metabolized primarily in the liver, where it undergoes phase I and phase II metabolic reactions . Enzymes such as cytochrome P450 play a crucial role in the oxidation and conjugation of this compound, leading to the formation of metabolites that are excreted from the body . These metabolic pathways can influence the compound’s bioavailability and therapeutic efficacy .
Transport and Distribution
The transport and distribution of this compound within cells and tissues are mediated by various transporters and binding proteins . The compound can cross cell membranes through passive diffusion and active transport mechanisms . Once inside the cells, this compound can bind to intracellular proteins, affecting its localization and accumulation . The distribution of the compound within tissues is influenced by factors such as blood flow, tissue permeability, and binding affinity to plasma proteins .
Subcellular Localization
The subcellular localization of this compound is critical for its activity and function. The compound has been found to localize in various cellular compartments, including the cytoplasm, mitochondria, and nucleus . Targeting signals and post-translational modifications can direct this compound to specific organelles, where it exerts its effects . For example, its localization in mitochondria is associated with the induction of apoptosis, while its presence in the nucleus can influence gene expression .
Preparation Methods
Synthetic Routes and Reaction Conditions: The synthesis of 2-Chloro-4-phenylthiazole typically involves the reaction of 2-aminothiazole with chlorinating agents. One common method is the reaction of 2-aminothiazole with phosphorus oxychloride (POCl3) in the presence of a base such as pyridine. The reaction is carried out under reflux conditions, leading to the formation of this compound .
Industrial Production Methods: Industrial production of this compound follows similar synthetic routes but on a larger scale. The process involves the use of large reactors and controlled conditions to ensure high yield and purity. The reaction parameters, such as temperature, pressure, and reaction time, are optimized to achieve efficient production .
Chemical Reactions Analysis
Types of Reactions: 2-Chloro-4-phenylthiazole undergoes various chemical reactions, including:
Substitution Reactions: The chlorine atom at the second position can be substituted with other nucleophiles, such as amines or thiols, leading to the formation of different derivatives.
Oxidation Reactions: The thiazole ring can undergo oxidation to form sulfoxides or sulfones.
Reduction Reactions: The compound can be reduced to form thiazolidines under specific conditions.
Common Reagents and Conditions:
Substitution Reactions: Reagents such as sodium hydride (NaH) or potassium carbonate (K2CO3) in polar aprotic solvents like dimethylformamide (DMF) are commonly used.
Oxidation Reactions: Oxidizing agents like hydrogen peroxide (H2O2) or m-chloroperbenzoic acid (m-CPBA) are employed.
Reduction Reactions: Reducing agents such as lithium aluminum hydride (LiAlH4) or sodium borohydride (NaBH4) are used.
Major Products Formed:
- Substituted thiazoles
- Thiazole sulfoxides and sulfones
- Thiazolidines
Scientific Research Applications
2-Chloro-4-phenylthiazole has a wide range of applications in scientific research:
Chemistry: It is used as a building block in the synthesis of various heterocyclic compounds and as a precursor for more complex molecules.
Biology: The compound exhibits antimicrobial, antifungal, and anticancer properties, making it a subject of interest in biological studies.
Medicine: Due to its biological activities, it is explored for potential therapeutic applications, including drug development for infectious diseases and cancer.
Industry: It is used in the production of dyes, pigments, and other industrial chemicals.
Comparison with Similar Compounds
- 2-Amino-4-phenylthiazole
- 2-Methyl-4-phenylthiazole
- 2-Bromo-4-phenylthiazole
Comparison: 2-Chloro-4-phenylthiazole is unique due to the presence of the chlorine atom, which imparts distinct reactivity and biological properties. Compared to 2-Amino-4-phenylthiazole, the chloro derivative is more reactive in substitution reactions. The methyl and bromo derivatives exhibit different electronic and steric effects, influencing their chemical behavior and biological activities .
Properties
IUPAC Name |
2-chloro-4-phenyl-1,3-thiazole | |
---|---|---|
Source | PubChem | |
URL | https://pubchem.ncbi.nlm.nih.gov | |
Description | Data deposited in or computed by PubChem | |
InChI |
InChI=1S/C9H6ClNS/c10-9-11-8(6-12-9)7-4-2-1-3-5-7/h1-6H | |
Source | PubChem | |
URL | https://pubchem.ncbi.nlm.nih.gov | |
Description | Data deposited in or computed by PubChem | |
InChI Key |
PNWMACLGSAOQCI-UHFFFAOYSA-N | |
Source | PubChem | |
URL | https://pubchem.ncbi.nlm.nih.gov | |
Description | Data deposited in or computed by PubChem | |
Canonical SMILES |
C1=CC=C(C=C1)C2=CSC(=N2)Cl | |
Source | PubChem | |
URL | https://pubchem.ncbi.nlm.nih.gov | |
Description | Data deposited in or computed by PubChem | |
Molecular Formula |
C9H6ClNS | |
Source | PubChem | |
URL | https://pubchem.ncbi.nlm.nih.gov | |
Description | Data deposited in or computed by PubChem | |
DSSTOX Substance ID |
DTXSID60375726 | |
Record name | 2-Chloro-4-phenylthiazole | |
Source | EPA DSSTox | |
URL | https://comptox.epa.gov/dashboard/DTXSID60375726 | |
Description | DSSTox provides a high quality public chemistry resource for supporting improved predictive toxicology. | |
Molecular Weight |
195.67 g/mol | |
Source | PubChem | |
URL | https://pubchem.ncbi.nlm.nih.gov | |
Description | Data deposited in or computed by PubChem | |
CAS No. |
1826-23-9 | |
Record name | 2-Chloro-4-phenylthiazole | |
Source | EPA DSSTox | |
URL | https://comptox.epa.gov/dashboard/DTXSID60375726 | |
Description | DSSTox provides a high quality public chemistry resource for supporting improved predictive toxicology. | |
Record name | 2-Chloro-4-phenylthiazole | |
Source | European Chemicals Agency (ECHA) | |
URL | https://echa.europa.eu/information-on-chemicals | |
Description | The European Chemicals Agency (ECHA) is an agency of the European Union which is the driving force among regulatory authorities in implementing the EU's groundbreaking chemicals legislation for the benefit of human health and the environment as well as for innovation and competitiveness. | |
Explanation | Use of the information, documents and data from the ECHA website is subject to the terms and conditions of this Legal Notice, and subject to other binding limitations provided for under applicable law, the information, documents and data made available on the ECHA website may be reproduced, distributed and/or used, totally or in part, for non-commercial purposes provided that ECHA is acknowledged as the source: "Source: European Chemicals Agency, http://echa.europa.eu/". Such acknowledgement must be included in each copy of the material. ECHA permits and encourages organisations and individuals to create links to the ECHA website under the following cumulative conditions: Links can only be made to webpages that provide a link to the Legal Notice page. | |
Synthesis routes and methods I
Procedure details
Synthesis routes and methods II
Procedure details
Synthesis routes and methods III
Procedure details
Retrosynthesis Analysis
AI-Powered Synthesis Planning: Our tool employs the Template_relevance Pistachio, Template_relevance Bkms_metabolic, Template_relevance Pistachio_ringbreaker, Template_relevance Reaxys, Template_relevance Reaxys_biocatalysis model, leveraging a vast database of chemical reactions to predict feasible synthetic routes.
One-Step Synthesis Focus: Specifically designed for one-step synthesis, it provides concise and direct routes for your target compounds, streamlining the synthesis process.
Accurate Predictions: Utilizing the extensive PISTACHIO, BKMS_METABOLIC, PISTACHIO_RINGBREAKER, REAXYS, REAXYS_BIOCATALYSIS database, our tool offers high-accuracy predictions, reflecting the latest in chemical research and data.
Strategy Settings
Precursor scoring | Relevance Heuristic |
---|---|
Min. plausibility | 0.01 |
Model | Template_relevance |
Template Set | Pistachio/Bkms_metabolic/Pistachio_ringbreaker/Reaxys/Reaxys_biocatalysis |
Top-N result to add to graph | 6 |
Feasible Synthetic Routes
Q1: How does 2-chloro-4-phenylthiazole (CPT) improve the performance of lithium-ion batteries?
A1: CPT functions as a film-forming additive in lithium-ion battery electrolytes. It decomposes at a specific voltage range (1100 mV to 1300 mV vs. Li/Li) [], forming a protective layer known as the Solid Electrolyte Interphase (SEI) on the surface of the graphite anode. This SEI layer is crucial for battery performance as it prevents further decomposition of the electrolyte, thus enhancing the battery's cycle life and stability.
Q2: What are the advantages of using CPT over conventional film-forming additives like vinylene carbonate (VC)?
A2: CPT offers a significant advantage over VC in terms of its decomposition potential. While VC decomposes at the edge of the potential window of carbonate-based electrolytes, CPT decomposes at a lower potential (1100 mV to 1300 mV vs. Li/Li) []. This earlier decomposition allows for SEI formation before reaching the critical potential window, leading to a more robust and effective protective layer on the anode.
Q3: What research methods were used to investigate the properties of CPT as a film-forming additive?
A3: The research paper utilized cyclovoltammetry and constant current cycling to evaluate the film-forming capabilities of CPT []. These electrochemical techniques allowed the researchers to analyze the decomposition behavior of CPT and its impact on the formation and stability of the SEI layer on the graphite anode.
Disclaimer and Information on In-Vitro Research Products
Please be aware that all articles and product information presented on BenchChem are intended solely for informational purposes. The products available for purchase on BenchChem are specifically designed for in-vitro studies, which are conducted outside of living organisms. In-vitro studies, derived from the Latin term "in glass," involve experiments performed in controlled laboratory settings using cells or tissues. It is important to note that these products are not categorized as medicines or drugs, and they have not received approval from the FDA for the prevention, treatment, or cure of any medical condition, ailment, or disease. We must emphasize that any form of bodily introduction of these products into humans or animals is strictly prohibited by law. It is essential to adhere to these guidelines to ensure compliance with legal and ethical standards in research and experimentation.