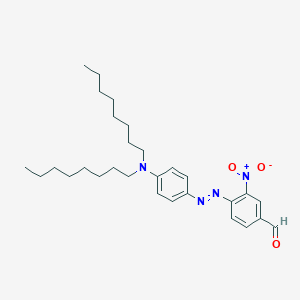
Bisulfite ionophore
Overview
Description
Bisulfite ionophore is a chemical species that facilitates the transport of bisulfite ions across cell membranes. Ionophores are generally lipid-soluble entities that can transport ions through hydrophobic membranes, such as those found in living cells or synthetic vesicles. Bisulfite ionophores are particularly useful in various analytical and industrial applications due to their ability to selectively bind and transport bisulfite ions.
Preparation Methods
Synthetic Routes and Reaction Conditions
The preparation of bisulfite ionophores typically involves the synthesis of organic compounds that can selectively bind bisulfite ions. These compounds are often incorporated into polymer membranes to create ion-selective electrodes. The synthetic route generally includes:
Synthesis of the Ionophore: Organic synthesis techniques are used to create the ionophore molecule. This may involve multiple steps, including the formation of specific functional groups that can interact with bisulfite ions.
Membrane Incorporation: The synthesized ionophore is then incorporated into a polymer membrane, such as polyvinyl chloride, along with plasticizers and additives to provide the membrane with the properties of a liquid phase.
Industrial Production Methods
In industrial settings, the production of bisulfite ionophores involves large-scale organic synthesis followed by membrane fabrication. The process is optimized for high yield and purity, ensuring that the ionophores are free from impurities that could affect their performance in analytical applications .
Chemical Reactions Analysis
Types of Reactions
Bisulfite ionophores primarily undergo complexation reactions with bisulfite ions. These reactions are characterized by the reversible binding of the ionophore to the bisulfite ion, facilitating its transport across membranes.
Common Reagents and Conditions
Reagents: Organic solvents, polymer membranes, plasticizers, and additives.
Major Products
The primary product of these reactions is the ionophore-bisulfite complex, which can be used in various analytical applications to detect and quantify bisulfite ions .
Scientific Research Applications
Bisulfite ionophores have a wide range of applications in scientific research:
Analytical Chemistry: Used in ion-selective electrodes for the detection and quantification of bisulfite ions in environmental and biological samples.
Environmental Monitoring: Employed in sensors to monitor bisulfite levels in water and air samples.
Medical Diagnostics: Utilized in diagnostic devices to measure bisulfite concentrations in physiological fluids.
Industrial Processes: Applied in process control to monitor bisulfite levels in various industrial processes.
Mechanism of Action
Bisulfite ionophores facilitate the transport of bisulfite ions across biological membranes through passive transport. The ionophore binds to the bisulfite ion, shielding its charge and allowing it to pass through the hydrophobic interior of the membrane. This process is driven by the concentration gradient of the bisulfite ion across the membrane .
Comparison with Similar Compounds
Similar Compounds
Valinomycin: A potassium ionophore that selectively binds and transports potassium ions.
Monensin: A sodium ionophore used in various biological applications.
Crown Ethers: Synthetic ionophores that can transport various cations, including potassium and sodium.
Uniqueness
Bisulfite ionophores are unique in their selectivity for bisulfite ions, making them particularly useful in applications where the detection and quantification of bisulfite are critical. Unlike other ionophores that may transport a range of cations, bisulfite ionophores are specifically designed to interact with bisulfite ions, providing high selectivity and sensitivity .
Properties
IUPAC Name |
4-[[4-(dioctylamino)phenyl]diazenyl]-3-nitrobenzaldehyde | |
---|---|---|
Source | PubChem | |
URL | https://pubchem.ncbi.nlm.nih.gov | |
Description | Data deposited in or computed by PubChem | |
InChI |
InChI=1S/C29H42N4O3/c1-3-5-7-9-11-13-21-32(22-14-12-10-8-6-4-2)27-18-16-26(17-19-27)30-31-28-20-15-25(24-34)23-29(28)33(35)36/h15-20,23-24H,3-14,21-22H2,1-2H3 | |
Source | PubChem | |
URL | https://pubchem.ncbi.nlm.nih.gov | |
Description | Data deposited in or computed by PubChem | |
InChI Key |
GOBXXSMKFPJVEM-UHFFFAOYSA-N | |
Source | PubChem | |
URL | https://pubchem.ncbi.nlm.nih.gov | |
Description | Data deposited in or computed by PubChem | |
Canonical SMILES |
CCCCCCCCN(CCCCCCCC)C1=CC=C(C=C1)N=NC2=C(C=C(C=C2)C=O)[N+](=O)[O-] | |
Source | PubChem | |
URL | https://pubchem.ncbi.nlm.nih.gov | |
Description | Data deposited in or computed by PubChem | |
Molecular Formula |
C29H42N4O3 | |
Source | PubChem | |
URL | https://pubchem.ncbi.nlm.nih.gov | |
Description | Data deposited in or computed by PubChem | |
DSSTOX Substance ID |
DTXSID60585040 | |
Record name | 4-{(E)-[4-(Dioctylamino)phenyl]diazenyl}-3-nitrobenzaldehyde | |
Source | EPA DSSTox | |
URL | https://comptox.epa.gov/dashboard/DTXSID60585040 | |
Description | DSSTox provides a high quality public chemistry resource for supporting improved predictive toxicology. | |
Molecular Weight |
494.7 g/mol | |
Source | PubChem | |
URL | https://pubchem.ncbi.nlm.nih.gov | |
Description | Data deposited in or computed by PubChem | |
CAS No. |
497151-94-7 | |
Record name | 4-{(E)-[4-(Dioctylamino)phenyl]diazenyl}-3-nitrobenzaldehyde | |
Source | EPA DSSTox | |
URL | https://comptox.epa.gov/dashboard/DTXSID60585040 | |
Description | DSSTox provides a high quality public chemistry resource for supporting improved predictive toxicology. | |
Retrosynthesis Analysis
AI-Powered Synthesis Planning: Our tool employs the Template_relevance Pistachio, Template_relevance Bkms_metabolic, Template_relevance Pistachio_ringbreaker, Template_relevance Reaxys, Template_relevance Reaxys_biocatalysis model, leveraging a vast database of chemical reactions to predict feasible synthetic routes.
One-Step Synthesis Focus: Specifically designed for one-step synthesis, it provides concise and direct routes for your target compounds, streamlining the synthesis process.
Accurate Predictions: Utilizing the extensive PISTACHIO, BKMS_METABOLIC, PISTACHIO_RINGBREAKER, REAXYS, REAXYS_BIOCATALYSIS database, our tool offers high-accuracy predictions, reflecting the latest in chemical research and data.
Strategy Settings
Precursor scoring | Relevance Heuristic |
---|---|
Min. plausibility | 0.01 |
Model | Template_relevance |
Template Set | Pistachio/Bkms_metabolic/Pistachio_ringbreaker/Reaxys/Reaxys_biocatalysis |
Top-N result to add to graph | 6 |
Feasible Synthetic Routes
Disclaimer and Information on In-Vitro Research Products
Please be aware that all articles and product information presented on BenchChem are intended solely for informational purposes. The products available for purchase on BenchChem are specifically designed for in-vitro studies, which are conducted outside of living organisms. In-vitro studies, derived from the Latin term "in glass," involve experiments performed in controlled laboratory settings using cells or tissues. It is important to note that these products are not categorized as medicines or drugs, and they have not received approval from the FDA for the prevention, treatment, or cure of any medical condition, ailment, or disease. We must emphasize that any form of bodily introduction of these products into humans or animals is strictly prohibited by law. It is essential to adhere to these guidelines to ensure compliance with legal and ethical standards in research and experimentation.