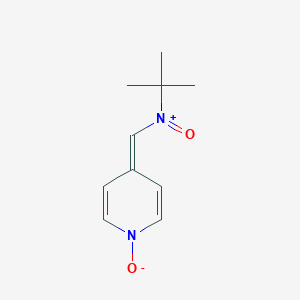
POBN
- Click on QUICK INQUIRY to receive a quote from our team of experts.
- With the quality product at a COMPETITIVE price, you can focus more on your research.
Overview
Description
α-(4-Pyridyl N-oxide)-N-tert-butylnitrone, commonly referred to as POBN, is a chemical compound with the molecular formula C₁₀H₁₄N₂O₂ and a molecular weight of 194.23 g/mol . It is a spin trap reagent used to detect free radicals, particularly hydroxyl radicals, in various biological and chemical systems .
Mechanism of Action
Target of Action
The primary targets of POBN are free radicals . Free radicals are unstable atoms that can damage cells, causing illness and aging. They are generated in the body due to various biochemical reactions and external factors like radiation and pollution.
Mode of Action
This compound acts as a spin-trapping agent . It interacts with free radicals, specifically 1-hydroxyethyl radicals , and forms a stable complex known as a spin adduct . This interaction helps in neutralizing the damaging effects of free radicals.
Biochemical Pathways
The formation of the spin adduct occurs through the reaction of this compound with 1-hydroxyethyl radicals . This reaction is part of the body’s antioxidant defense mechanism, which helps in neutralizing harmful free radicals. The formation of the spin adduct can be detected in the bile when high concentrations of this compound are achieved through intravenous injection .
Pharmacokinetics
The pharmacokinetics of this compound involve its intravenous administration, which leads to high concentrations of the compound in the body . This allows for the effective trapping of free radicals. The spin adducts formed are detectable in the bile .
Result of Action
The result of this compound’s action is the formation of a stable spin adduct with 1-hydroxyethyl radicals . This helps in reducing the damaging effects of these radicals on the body’s cells. The effectiveness of this compound in trapping these radicals can be observed through the detection of the spin adduct in the bile .
Action Environment
The action of this compound can be influenced by various environmental factors. For instance, the presence of alcohol can enhance the formation of 1-hydroxyethyl radical spin adducts of this compound . Furthermore, the effectiveness of this compound in trapping free radicals can be influenced by the concentration of the compound achieved in the body .
Biochemical Analysis
Biochemical Properties
Alpha-(4-Pyridyl-1-oxide)-N-tert-butylnitrone has been shown to interact with 1-hydroxyethyl radicals . This interaction is crucial in the detection of these radicals in vivo .
Cellular Effects
It is known that it can interact with radicals within cells, which may have implications for cellular function .
Molecular Mechanism
Alpha-(4-Pyridyl-1-oxide)-N-tert-butylnitrone works by trapping 1-hydroxyethyl radicals . This allows for the detection of these radicals, which can be crucial in understanding certain biochemical processes .
Preparation Methods
Synthetic Routes and Reaction Conditions
The synthesis of α-(4-Pyridyl N-oxide)-N-tert-butylnitrone typically involves the reaction of 4-pyridinecarboxaldehyde N-oxide with tert-butylamine in the presence of an oxidizing agent . The reaction is carried out under controlled conditions to ensure the formation of the desired product. The reaction conditions often include a solvent such as methanol or ethanol and a temperature range of 0-25°C .
Industrial Production Methods
Industrial production of α-(4-Pyridyl N-oxide)-N-tert-butylnitrone follows similar synthetic routes but on a larger scale. The process involves the use of large reactors and precise control of reaction parameters to ensure high yield and purity of the product . The compound is then purified using techniques such as recrystallization or chromatography to achieve the desired purity level .
Chemical Reactions Analysis
Types of Reactions
α-(4-Pyridyl N-oxide)-N-tert-butylnitrone undergoes various chemical reactions, including:
Oxidation: It can react with oxidizing agents to form oxidized products.
Reduction: It can be reduced to form the corresponding amine.
Substitution: It can undergo substitution reactions with nucleophiles.
Common Reagents and Conditions
Oxidation: Common oxidizing agents include hydrogen peroxide and peroxides.
Reduction: Reducing agents such as sodium borohydride or lithium aluminum hydride are used.
Substitution: Nucleophiles such as amines or thiols can be used in substitution reactions.
Major Products Formed
The major products formed from these reactions depend on the specific reagents and conditions used. For example, oxidation of α-(4-Pyridyl N-oxide)-N-tert-butylnitrone can lead to the formation of hydroxyl radical adducts .
Scientific Research Applications
α-(4-Pyridyl N-oxide)-N-tert-butylnitrone is widely used in scientific research due to its ability to trap free radicals. Some of its applications include:
Chemistry: Used as a spin trap reagent to detect and study free radicals in various chemical reactions.
Biology: Employed in studies of oxidative stress and free radical formation in biological systems.
Industry: Applied in the analysis of lipid peroxidation and oxidative stability of oils and fats.
Comparison with Similar Compounds
α-(4-Pyridyl N-oxide)-N-tert-butylnitrone is similar to other spin trap reagents such as α-phenyl-N-tert-butylnitrone (PBN) and 5,5-dimethyl-1-pyrroline-N-oxide (DMPO) . it has unique properties that make it particularly useful in certain applications:
Higher water solubility: Compared to PBN, α-(4-Pyridyl N-oxide)-N-tert-butylnitrone is more water-soluble, making it suitable for aqueous systems.
Specificity: It has a higher specificity for certain free radicals, such as hydroxyl radicals.
List of Similar Compounds
- α-Phenyl-N-tert-butylnitrone (PBN)
- 5,5-Dimethyl-1-pyrroline-N-oxide (DMPO)
- α-(4-Pyridyl N-oxide)-N-tert-butylnitrone (POBN)
Properties
CAS No. |
66893-81-0 |
---|---|
Molecular Formula |
C10H14N2O2 |
Molecular Weight |
194.23 g/mol |
IUPAC Name |
N-tert-butyl-1-(1-oxidopyridin-1-ium-4-yl)methanimine oxide |
InChI |
InChI=1S/C10H14N2O2/c1-10(2,3)12(14)8-9-4-6-11(13)7-5-9/h4-8H,1-3H3/b12-8+ |
InChI Key |
RNRMWTCECDHNQU-XYOKQWHBSA-N |
Isomeric SMILES |
CC(C)(C)/[N+](=C\C1=CC=[N+](C=C1)[O-])/[O-] |
SMILES |
CC(C)(C)[N+](=CC1=CC=[N+](C=C1)[O-])[O-] |
Canonical SMILES |
CC(C)(C)[N+](=CC1=CC=[N+](C=C1)[O-])[O-] |
Synonyms |
4-POBN 4-PyOBN 4-pyridyl-1-oxide-T-butylnitrone alpha-(4-pyridyl-1-oxide)-N-tert-butylnitrone POBN |
Origin of Product |
United States |
Retrosynthesis Analysis
AI-Powered Synthesis Planning: Our tool employs the Template_relevance Pistachio, Template_relevance Bkms_metabolic, Template_relevance Pistachio_ringbreaker, Template_relevance Reaxys, Template_relevance Reaxys_biocatalysis model, leveraging a vast database of chemical reactions to predict feasible synthetic routes.
One-Step Synthesis Focus: Specifically designed for one-step synthesis, it provides concise and direct routes for your target compounds, streamlining the synthesis process.
Accurate Predictions: Utilizing the extensive PISTACHIO, BKMS_METABOLIC, PISTACHIO_RINGBREAKER, REAXYS, REAXYS_BIOCATALYSIS database, our tool offers high-accuracy predictions, reflecting the latest in chemical research and data.
Strategy Settings
Precursor scoring | Relevance Heuristic |
---|---|
Min. plausibility | 0.01 |
Model | Template_relevance |
Template Set | Pistachio/Bkms_metabolic/Pistachio_ringbreaker/Reaxys/Reaxys_biocatalysis |
Top-N result to add to graph | 6 |
Feasible Synthetic Routes
Disclaimer and Information on In-Vitro Research Products
Please be aware that all articles and product information presented on BenchChem are intended solely for informational purposes. The products available for purchase on BenchChem are specifically designed for in-vitro studies, which are conducted outside of living organisms. In-vitro studies, derived from the Latin term "in glass," involve experiments performed in controlled laboratory settings using cells or tissues. It is important to note that these products are not categorized as medicines or drugs, and they have not received approval from the FDA for the prevention, treatment, or cure of any medical condition, ailment, or disease. We must emphasize that any form of bodily introduction of these products into humans or animals is strictly prohibited by law. It is essential to adhere to these guidelines to ensure compliance with legal and ethical standards in research and experimentation.