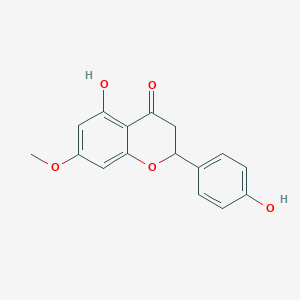
5-Hydroxy-2-(4-hydroxyphenyl)-7-methoxychroman-4-one
Overview
Description
Mechanism of Action
Target of Action
Sakuranetin, a naturally derived 7-O-methylated flavonoid , primarily targets the 3-hydroxyacyl-[acyl-carrier-protein] dehydratase FabZ in Helicobacter pylori . It also targets the clathrin-mediated endocytosis (CME) pathway in rice cells .
Mode of Action
Sakuranetin interacts with its targets and results in significant changes. It attenuates the endocytosis of various plasma membrane proteins and the fungal effector PWL2 . The inhibitory effect of sakuranetin is dosage-dependent and targets the CME pathway . This interaction leads to a decrease in the accumulation of the avirulence protein AvrCO39, which is taken up into rice cells by Magnaporthe oryzae .
Biochemical Pathways
Sakuranetin affects the biochemical pathways related to endocytosis. It attenuates the CME pathway, which is involved in the uptake of various plasma membrane proteins and fungal effectors into rice cells . This attenuation of CME by sakuranetin is sufficient to convey resistance against rice blast .
Pharmacokinetics
It is known that sakuranetin is a small molecule , which suggests that it may have good bioavailability
Result of Action
The molecular and cellular effects of sakuranetin’s action are diverse. It has been reported to have antioxidant, anti-inflammatory, antimycobacterial, antiviral, antifungal, antileishmanial, antitrypanosomal, glucose uptake stimulation, neuroprotective, antimelanogenic, and antitumor properties . In addition, sakuranetin has been shown to prevent pulmonary inflammation induced by air pollution .
Action Environment
Environmental factors can influence the action, efficacy, and stability of sakuranetin. For instance, in plants, sakuranetin functions as a phytoalexin and is released in response to different abiotic and biotic stresses such as UV-irradiation, jasmonic acid, cupric chloride, L-methionine, and the phytotoxin coronatine
Biochemical Analysis
Biochemical Properties
Sakuranetin plays a crucial role in various biochemical reactions. It interacts with several enzymes, proteins, and other biomolecules. For instance, sakuranetin has been shown to inhibit the activity of cyclooxygenase-2 (COX-2), an enzyme involved in the inflammatory response . Additionally, sakuranetin interacts with nuclear factor kappa-light-chain-enhancer of activated B cells (NF-κB), a protein complex that controls the transcription of DNA, cytokine production, and cell survival . These interactions highlight the anti-inflammatory properties of sakuranetin.
Cellular Effects
Sakuranetin exerts significant effects on various types of cells and cellular processes. It influences cell function by modulating cell signaling pathways, gene expression, and cellular metabolism. For example, sakuranetin has been found to inhibit the proliferation of cancer cells by inducing apoptosis and cell cycle arrest . It also affects the expression of genes involved in inflammation and immune response, thereby exhibiting its anti-inflammatory and immunomodulatory effects . Furthermore, sakuranetin impacts cellular metabolism by modulating the activity of enzymes involved in metabolic pathways .
Molecular Mechanism
The molecular mechanism of sakuranetin involves several binding interactions with biomolecules, enzyme inhibition or activation, and changes in gene expression. Sakuranetin binds to the active site of COX-2, thereby inhibiting its activity and reducing the production of pro-inflammatory mediators . It also interacts with NF-κB, preventing its translocation to the nucleus and subsequent activation of inflammatory genes . Additionally, sakuranetin modulates the expression of genes involved in apoptosis, cell cycle regulation, and immune response . These molecular interactions underpin the pharmacological effects of sakuranetin.
Temporal Effects in Laboratory Settings
In laboratory settings, the effects of sakuranetin change over time. Sakuranetin exhibits stability under various conditions, but its degradation can occur under extreme pH or temperature . Long-term studies have shown that sakuranetin maintains its anti-inflammatory and anti-tumor effects over extended periods . Prolonged exposure to sakuranetin may lead to adaptive cellular responses, such as upregulation of detoxifying enzymes . These temporal effects highlight the importance of optimizing sakuranetin’s stability and dosage for therapeutic applications.
Dosage Effects in Animal Models
The effects of sakuranetin vary with different dosages in animal models. At low doses, sakuranetin exhibits anti-inflammatory and immunomodulatory effects without significant toxicity . At higher doses, sakuranetin may cause adverse effects, such as hepatotoxicity and gastrointestinal disturbances . Threshold effects have been observed, where sakuranetin’s efficacy plateaus beyond a certain dosage . These findings underscore the need for careful dosage optimization in preclinical and clinical studies.
Metabolic Pathways
Sakuranetin is involved in several metabolic pathways, interacting with enzymes and cofactors. It undergoes biotransformation through demethylation to naringenin, followed by aromatic hydroxylation to eriodictyol . These metabolic conversions are catalyzed by enzymes such as cytochrome P450 . Sakuranetin also affects metabolic flux by modulating the activity of key enzymes in metabolic pathways . These interactions highlight the complex metabolic fate of sakuranetin in biological systems.
Transport and Distribution
Sakuranetin is transported and distributed within cells and tissues through various mechanisms. It interacts with transporters and binding proteins that facilitate its cellular uptake and distribution . Sakuranetin’s localization and accumulation are influenced by its physicochemical properties, such as lipophilicity and molecular size . These factors determine its bioavailability and therapeutic efficacy in different tissues .
Subcellular Localization
The subcellular localization of sakuranetin plays a crucial role in its activity and function. Sakuranetin is primarily localized in the cytoplasm, where it interacts with enzymes and signaling proteins . It may also be targeted to specific compartments or organelles through post-translational modifications or targeting signals . These localization patterns influence sakuranetin’s pharmacological effects and therapeutic potential.
Preparation Methods
Synthetic Routes and Reaction Conditions
The synthesis of sakuranetin can be achieved through several methods. One common approach involves the methylation of naringenin, a flavanone, using methyl iodide in the presence of a base such as potassium carbonate. The reaction is typically carried out in an organic solvent like acetone at room temperature .
Industrial Production Methods
Industrial production of sakuranetin may involve the extraction from plant sources, such as rice plants, where it is naturally found. The extraction process includes solvent extraction, purification, and crystallization to obtain the pure compound .
Chemical Reactions Analysis
Types of Reactions
Sakuranetin undergoes various chemical reactions, including:
Oxidation: Sakuranetin can be oxidized to form sakuranetin-7-O-glucoside.
Reduction: Reduction of sakuranetin can yield dihydrosakuranetin.
Common Reagents and Conditions
Oxidation: Common oxidizing agents include hydrogen peroxide and potassium permanganate.
Reduction: Reducing agents like sodium borohydride are used.
Substitution: Reagents such as halogens (chlorine, bromine) and alkylating agents are employed.
Major Products Formed
Oxidation: Sakuranetin-7-O-glucoside
Reduction: Dihydrosakuranetin
Substitution: Various substituted derivatives depending on the reagents used.
Scientific Research Applications
Chemistry: Used as a probe in chemical reactions to study reaction mechanisms and pathways.
Biology: Investigated for its role in plant defense mechanisms and its ability to modulate biological processes.
Medicine: Explored for its anti-inflammatory, antioxidant, and antimicrobial properties, making it a potential candidate for drug development.
Comparison with Similar Compounds
Similar Compounds
Naringenin: A flavanone similar to sakuranetin but lacks the methoxy group at the 7-position.
Hesperetin: Another flavanone with similar antioxidant properties but different structural features.
Apigenin: A flavone with similar anti-inflammatory properties but a different core structure.
Uniqueness of Sakuranetin
Sakuranetin’s unique structural feature is the methoxy group at the 7-position, which contributes to its distinct biological activities and makes it a valuable compound for various applications .
Properties
IUPAC Name |
5-hydroxy-2-(4-hydroxyphenyl)-7-methoxy-2,3-dihydrochromen-4-one | |
---|---|---|
Source | PubChem | |
URL | https://pubchem.ncbi.nlm.nih.gov | |
Description | Data deposited in or computed by PubChem | |
InChI |
InChI=1S/C16H14O5/c1-20-11-6-12(18)16-13(19)8-14(21-15(16)7-11)9-2-4-10(17)5-3-9/h2-7,14,17-18H,8H2,1H3 | |
Source | PubChem | |
URL | https://pubchem.ncbi.nlm.nih.gov | |
Description | Data deposited in or computed by PubChem | |
InChI Key |
DJOJDHGQRNZXQQ-UHFFFAOYSA-N | |
Source | PubChem | |
URL | https://pubchem.ncbi.nlm.nih.gov | |
Description | Data deposited in or computed by PubChem | |
Canonical SMILES |
COC1=CC(=C2C(=O)CC(OC2=C1)C3=CC=C(C=C3)O)O | |
Source | PubChem | |
URL | https://pubchem.ncbi.nlm.nih.gov | |
Description | Data deposited in or computed by PubChem | |
Molecular Formula |
C16H14O5 | |
Source | PubChem | |
URL | https://pubchem.ncbi.nlm.nih.gov | |
Description | Data deposited in or computed by PubChem | |
DSSTOX Substance ID |
DTXSID10874774 | |
Record name | Sakuranetin | |
Source | EPA DSSTox | |
URL | https://comptox.epa.gov/dashboard/DTXSID10874774 | |
Description | DSSTox provides a high quality public chemistry resource for supporting improved predictive toxicology. | |
Molecular Weight |
286.28 g/mol | |
Source | PubChem | |
URL | https://pubchem.ncbi.nlm.nih.gov | |
Description | Data deposited in or computed by PubChem | |
CAS No. |
2957-21-3 | |
Record name | Sakuranetin | |
Source | DTP/NCI | |
URL | https://dtp.cancer.gov/dtpstandard/servlet/dwindex?searchtype=NSC&outputformat=html&searchlist=407228 | |
Description | The NCI Development Therapeutics Program (DTP) provides services and resources to the academic and private-sector research communities worldwide to facilitate the discovery and development of new cancer therapeutic agents. | |
Explanation | Unless otherwise indicated, all text within NCI products is free of copyright and may be reused without our permission. Credit the National Cancer Institute as the source. | |
Record name | Sakuranetin | |
Source | EPA DSSTox | |
URL | https://comptox.epa.gov/dashboard/DTXSID10874774 | |
Description | DSSTox provides a high quality public chemistry resource for supporting improved predictive toxicology. | |
Record name | 4',5-dihydroxy-7-methoxyflavone | |
Source | European Chemicals Agency (ECHA) | |
URL | https://echa.europa.eu/substance-information/-/substanceinfo/100.019.073 | |
Description | The European Chemicals Agency (ECHA) is an agency of the European Union which is the driving force among regulatory authorities in implementing the EU's groundbreaking chemicals legislation for the benefit of human health and the environment as well as for innovation and competitiveness. | |
Explanation | Use of the information, documents and data from the ECHA website is subject to the terms and conditions of this Legal Notice, and subject to other binding limitations provided for under applicable law, the information, documents and data made available on the ECHA website may be reproduced, distributed and/or used, totally or in part, for non-commercial purposes provided that ECHA is acknowledged as the source: "Source: European Chemicals Agency, http://echa.europa.eu/". Such acknowledgement must be included in each copy of the material. ECHA permits and encourages organisations and individuals to create links to the ECHA website under the following cumulative conditions: Links can only be made to webpages that provide a link to the Legal Notice page. | |
Retrosynthesis Analysis
AI-Powered Synthesis Planning: Our tool employs the Template_relevance Pistachio, Template_relevance Bkms_metabolic, Template_relevance Pistachio_ringbreaker, Template_relevance Reaxys, Template_relevance Reaxys_biocatalysis model, leveraging a vast database of chemical reactions to predict feasible synthetic routes.
One-Step Synthesis Focus: Specifically designed for one-step synthesis, it provides concise and direct routes for your target compounds, streamlining the synthesis process.
Accurate Predictions: Utilizing the extensive PISTACHIO, BKMS_METABOLIC, PISTACHIO_RINGBREAKER, REAXYS, REAXYS_BIOCATALYSIS database, our tool offers high-accuracy predictions, reflecting the latest in chemical research and data.
Strategy Settings
Precursor scoring | Relevance Heuristic |
---|---|
Min. plausibility | 0.01 |
Model | Template_relevance |
Template Set | Pistachio/Bkms_metabolic/Pistachio_ringbreaker/Reaxys/Reaxys_biocatalysis |
Top-N result to add to graph | 6 |
Feasible Synthetic Routes
Disclaimer and Information on In-Vitro Research Products
Please be aware that all articles and product information presented on BenchChem are intended solely for informational purposes. The products available for purchase on BenchChem are specifically designed for in-vitro studies, which are conducted outside of living organisms. In-vitro studies, derived from the Latin term "in glass," involve experiments performed in controlled laboratory settings using cells or tissues. It is important to note that these products are not categorized as medicines or drugs, and they have not received approval from the FDA for the prevention, treatment, or cure of any medical condition, ailment, or disease. We must emphasize that any form of bodily introduction of these products into humans or animals is strictly prohibited by law. It is essential to adhere to these guidelines to ensure compliance with legal and ethical standards in research and experimentation.